The future of information storage devices: molecule-based magnets
Author: Tommaso Francese is a Ph.D. student (ITN-EJD-TCCM) at Universitat de Barcelona
Every year the amount of data produced is of the order of magnitude of the Avogadro’s constant, thus 6.028×1023. Let’s say that it is more or less the quantity of hydrogen atoms contained in 1 gram. This trend is supposed to increase even more in the next future. The point is: what might happen if the resources used for manufacturing the common storage devices disappear? So, in this sense we are now investigating a new family of compounds that can be exploited for replacing these elements, and moreover, create cheaper devices. What else?
These compounds are completely organic. It means that: a) the resources are available everywhere; b) the resources are extremely cheap; c) there is a great versatility for synthesizing the different compounds.
Moreover, the added value of these organic compounds is the fact they present magnetic properties, like the ones present in an iron-based magnet. This is incredible if you think that we are talking about purely organic compounds.
Ok, some more details: the family we are investigating is the Dithiazolyl-based (DTA) compounds. It means that all the compound belonging to this big family ( more than 40 different compounds) share the same moiety in which one nitrogen atom is in between of two sulfur atoms: – – S—N•—S – –
The nitrogen N formally hosts the unpaired electron. That’s why we can call these compounds “radicals”. Radicals are extremely interesting systems with an extremely high reactivity. They could be atoms, ions or molecules, like in our case. Radicals are interesting molecules for spintronics1. One more interesting feature is that some of the DTA-based compounds present bistability. Bistability refers to the co-existence of two stable phases/polymorphs within the same range of temperature, but above and below this range of temperature only one of two existing systems can exist. The correlation between magnetic properties and bistability is not so obvious. Up to now there is not a clear explanation why it occurs in some systems and not in others.
It is in this context that we are playing a key role, trying first to explain such unusual behavior, and secondly trying to unveil the basic mechanism that undergoes to these materials. In this sense the first system that was analyzed keeping in mind what discussed above was the so called TTTA2. Are you wondering why? Easy, it was extremely well characterized from an experimental point of view by means of X-Ray Powder Diffraction ( a technique that exploits the interference between crystal planes and light in order to determine the structure of the system investigated ), Electron Paramagnetic Resonance, a.k.a. EPR, used for analyzing particular species that present unpaired electrons, i.e. radicals, and measuring the so called Magnetic Susceptibility by changing the force felt upon a substance when a magnetic field gradient is applied3.
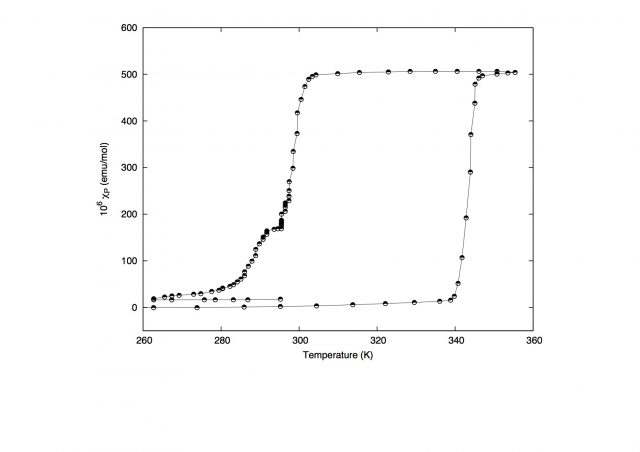
The structure of the TTTA is very interesting, being magnetic and bistable. The susceptibility curve, Figure 1, encompasses room temperature (25°C or 298K) and two stable polymorphs, one at Low Temperature (LT) and the other at High Temperature (HT). The LT polymorph is diamagnetic, it means that once a magnetic field is applied, the material will create an induced magnetic field in the opposite direction of the one externally applied.
On the other hand, the HT polymorph instead is paramagnetic. So, the final behavior is a balancing between the two magnetic responses. Moreover, from a structural point of view, the LT phase is triclinic with eclipsed -pairs alternated with -shifted pairs, (Figure 2- Left), while the HT phase is monoclinic, with uniform -stack pairs (Figure 2-Right).
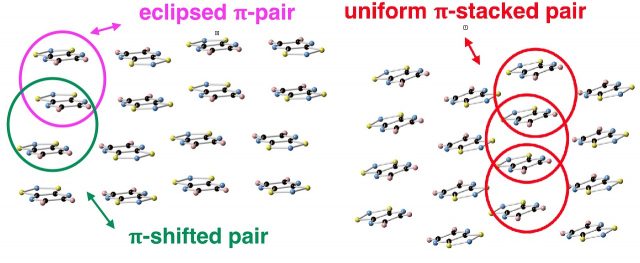
Why? That’s another very interesting point: there is a strong mutual correlation between the structural behavior and the magnetic response of the two different systems. All these points were used as a “stimuli” for developing a theoretical methodology in order to explain these features. The so called “STATIC” analysis allows you to get an overall picture of your system. It costs of 4 steps, and it’s name is First-Principles Bottom Up4.
The first step relates to the analysis of the bulk system resolve by X-Ray. Some softwares allows you to literally manipulate the crystal structure, even if it extremely complex. Once all the possible two-molecule cluster combinations have been selected from the bulk system (max. distance between molecules = 10 Angstroms), then we can proceed with the computation of the “magnetic interaction”. This point is fundamental, because it gives us the possibility to literally see which is the combination of molecules inside the crystal that present the strongest magnetic interaction. Let’s say that is look like to for an investigator to capture a thief and get his finger prints. Once completed, the second step is the reconstruction of the “magnetic skeleton” of the bulk system investigated, Figure 3. The third step is the accurate selection of some “minimal magnetic models”, fragments of the 3D magnetic topology previously defined, that, if correctly defined, should allow you to 1) rebuilt the infinite 3D magnetic topology of the original structure, and 2) if expanded along the 3 crystallographic axes (x,y and z), the related magnetic property should converge towards the experimental one. Once done, exploiting the power of Quantum Mechanics [5], we can compute the energy spectra of the different models. The energy spectrum is the “list” of all the possible energetic interaction, called eigenstates, between all the spin centers. This “list” is used in the fourth and last step to compute the magnetic susceptibility of the system investigated, by means of statistical mechanics formulas.
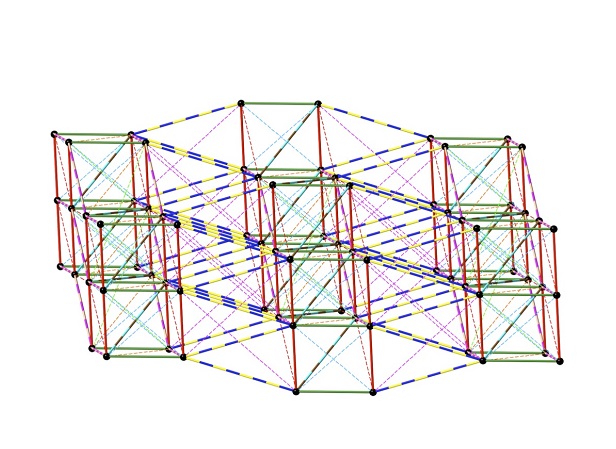
The power of this technique resides in the fact that it permits to describe a complex system, like TTTA, and computing a magnetic property of interest, that is a non-trivial stuff, in just 4 steps.
The bottomline is: 1) to be able to identify the two molecule cluster responsible for the magnetic behavior and 2) to reproduce the “static” view of the magnetic susceptibility.
Let’s move a little bit further: now we want to include also thermal fluctuations in our simulations. Possible? Yes! Things are quite far from being an easy deal, by the way here I provide a qualitative description of how it works. There some very specific codes designed in order to compute these particular kind of calculations. These computations are called Molecular Dynamics ( a.k.a. MD). In few words, we virtually couple a thermostat, a device that keeps temperature constant in the around of a precise value previously defined, to our bulk system. The simulation runs for a certain period, usually 10 picoseconds ( 1 ps = 10-12 s ), valid for Ab Initio MD, from which we can observe the dynamical evolution of the system of interest as a function of time and temperature.
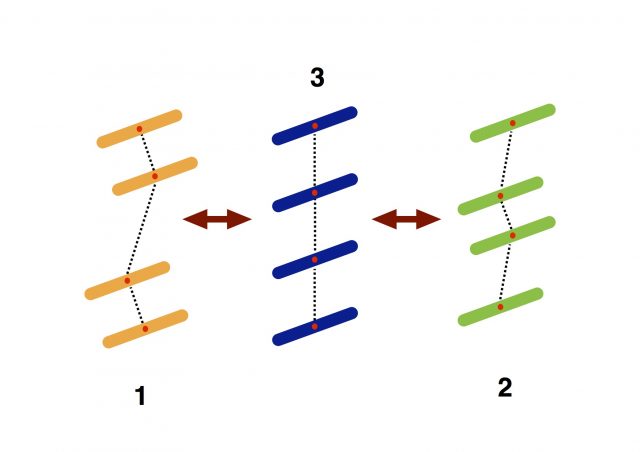
Thanks to this powerful means, it was possible to unveil that the HT polymorph is continuously “jumping” between two low energy configurations (1 and 2), via transition step 3 (Figure 4). This discovery was extremely important because, to know exactly how the material behaves as a function of temperature, get us the possibility to design a new system that exploit the related magnetic properties but possibly having one of the two possible configurations. The LT—>HT phase transition can be seen as a switch between the states 0 and 1, so acquiring/loosing information. This is the key potential for using this kind of molecules and system for the next generation of storage devices. On the other hand, these materials can find a potential applicability also for heat or photo sensor. In fact the “jump” from one polymorph to the other can also be induced by light. But this is another story!
References
- Wolf, S. A.; Chtchelkanova, A. Y.; Treger, D. M. (2006). “Spintronics – A retrospective and perspective”. IBM Journal of Research and Development. 50: 101. doi:10.1147/rd.501.0101 ↩
- Vela, Sergi, et al. Nature communications 5 (2014). ↩
- Ira N. Levine (1975). Molecular Spectroscopy. Wiley & Sons, Inc. p. 380 ↩
- Clarke, C.S et al (2010) Origin of the Magnetic Bistability in Molecule-Based Magnets: A First-Principles Bottom-Up Study of the TTTA Crystal J. Am. Chem. Soc. 2010, 132, 17817-17830 ↩
1 comment
[…] ¿Cómo seguir almacenando datos, que crecen a un ritmo exponencial, cuando nos quedemos sin las materias primas que usamos ahora? Tommaso Francese tiene una sugerencia en The future of information storage devices: molecule-based magnets. […]