Validating the existence of a new phase of matter, the exciton condensate
According to how the electronic band theory is usually explained, solids can be classified as insulators, semiconductors, or metals. But, actually, there is another kind of solid between semiconductors and metals, the semimetals. In insulators and semiconductors the filled valence band is separated from an empty conduction band by a band gap, in metals there is a partially filled conduction band and in semimetals we find a very small overlap between the bottom of the conduction band and the top of the valence band.
Semimetals could undergo, at sufficiently low temperatures, a phase transition into an insulating state. This state is described by excitons. A hole being a vacant electron position in the lattice structure of the solid, an exciton is a electron-hole pair that is bound in a manner analogous to the electron and proton in a hydrogen atom. These pairs form a so-called exciton condensate and the system is said to be in an excitonic insulating phase. The ground state of such a phase can be described with the help of a BCS-like theory, in analogy with the superconducting phase.
However, the coupling strength in an excitonic insulator is expected to be even weaker than in a superconductor. Furthermore, electron-hole recombination can be quite fast, thereby preventing the formation of the condensate. For these reasons the exciton condensate remains an elusive phase of matter.
Possible semimetal candidates suggested to undergo a transition to the excitonic insulating phase with an exciton condensate are transition-metal dichalcogenide TiSe2 and HgTe quantum well with a thickness of 20 nm. However, there is no conclusive evidence for an exciton condensate in such systems.
In addition to bulk semimetals, there have been several proposals to create an exciton condensate in systems with spatially separated electron and hole gases in order to reduce the electron-hole recombination rate. The exciton formation in such electron hole bilayers can be detected by Coulomb drag measurements. According to the theory, if the excitons form a condensate, one expects a discontinuity in the drag at the critical temperature and a divergence when the temperature approaches 0. However, the experimental evidence shows that, even if certain anomalies of the Coulomb drag as a function of temperature have been observed, it is hard to attribute them to the formation of an exciton condensate.
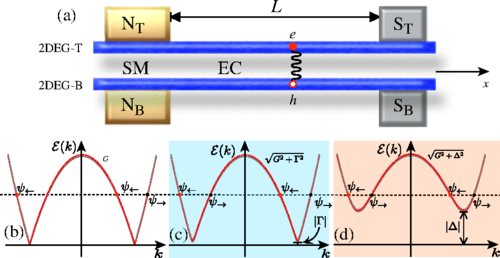
It is clear that additional type of measurements to validate the existence of an exciton condensate in electron-hole bilayers are needed. Now, Ikerbasque researcher Dario Bercioux (DIPC), Teun Klapwijk (Kavli Institute of Nanoscience) and F. Sebastián Bergeret (CFM; DIPC) suggest 1, instead of measuring the Coulomb drag, to perform a differential conductance measurement using normal and superconducting electrodes (see Figure 1) in order to unveil the presence of the excitonic gap directly.
The researchers theoretically study the electronic transport through an electron-hole bilayer in contact with normal and superconducting electrodes, assuming that the electron-hole bilayer hosts an exciton condensate.
They find that the transport properties of this junction are determined by the competition of different coherent reflection processes occurring at the interfaces with the normal and superconducting electrodes. As a consequence of this competition, the differential conductance has a minimum. The observation of this minimum in an electron-hole bilayer system would represent a unique hallmark of the presence of the exciton condensate.
Good candidates to check experimentally this proposal would be double bilayer-graphene systems separated by hexagonal boron nitrate. Actually, some results could be reinterpreted in the light of these results. Namely, the zero-bias peak observed in the differential resistance of a HgTe quantum well-superconductor junction could be a manifestation of an exciton condensate phase.
Author: César Tomé López is a science writer and the editor of Mapping Ignorance.
References
- D. Bercioux,T. M. Klapwijk, and F. S. Bergeret (2017) Transport Properties of an Electron-Hole Bilayer in Contact with a Superconductor Hybrid Junction Phys. Rev Lett. doi: 10.1103/PhysRevLett.119.067001 ↩