Matter manipulation with terahertz radiation
Terahertz (THz) radiation is all around us. For example, this page emits blackbody radiation mainly in the THz region (defined here from 0.3 THz to 30 THz). Because terahertz radiation begins at a wavelength of around one millimeter and proceeds into shorter wavelengths, it is sometimes known as the submillimeter band, and its radiation as submillimeter waves, especially in astronomy.
This band of electromagnetic radiation can be regarded either as microwave radio waves or far infrared. The technology for its generation and manipulation is in its infancy, that is why enginners talk about the Thz gap. One of the reasons is that THz radiation from traditional microwave sources is usually too weak to have any measurable impact on the properties of materials.
The situation drastically changed at the beginning of the 2000s, when the technique of optical rectification with phase matching in crystals lacking inversion symmetry such as lithium niobate was developed. This technological breakthrough enabled the development of table-top sources of single-cycle THz pulses with field strengths comparable to the intrinsic field strength in a wide range of strongly correlated materials. Consequently, it became possible to engineer new dynamic states of materials by modifying their intrinsic fields.
In condensed matter physics and in general, THz radiation is an efficient tool to investigate a multitude of low-energy excitations existing in the THz region. Important examples are resonances of phonons and plasmons (collective lattice and electron vibrations, respectively), spins, intersubband transitions (subbands are electronic energy bands formed in quantum wells, in which the electrons are confined in two directions), excitons (bound electron–hole pairs), macro-molecular vibrations and molecular rotations.
THz radiation is also a fine tool: the energy of THz photons matches the fine energy structure of the mentioned resonances. This offers clear benefits when compared to optical photons, as these often contain excessive energy relative to low-energy resonances. The extra energy from the optical photons is, for example, distributed as phonon excitations or hot electron distributions, which result in less control and unwanted temperature increase. THz radiation, on the other hand, can be used to specifically target the excitation of interest, opening the door to a controlled manipulation of reactions, processes, and properties of matter.
In addition to the collective excitations mentioned, several other systems can be driven by strong-field few-cycle THz pulses. The characteristic energy regions of various THz excitations and related examples of strong-field THz applications are presented in Figure 1.
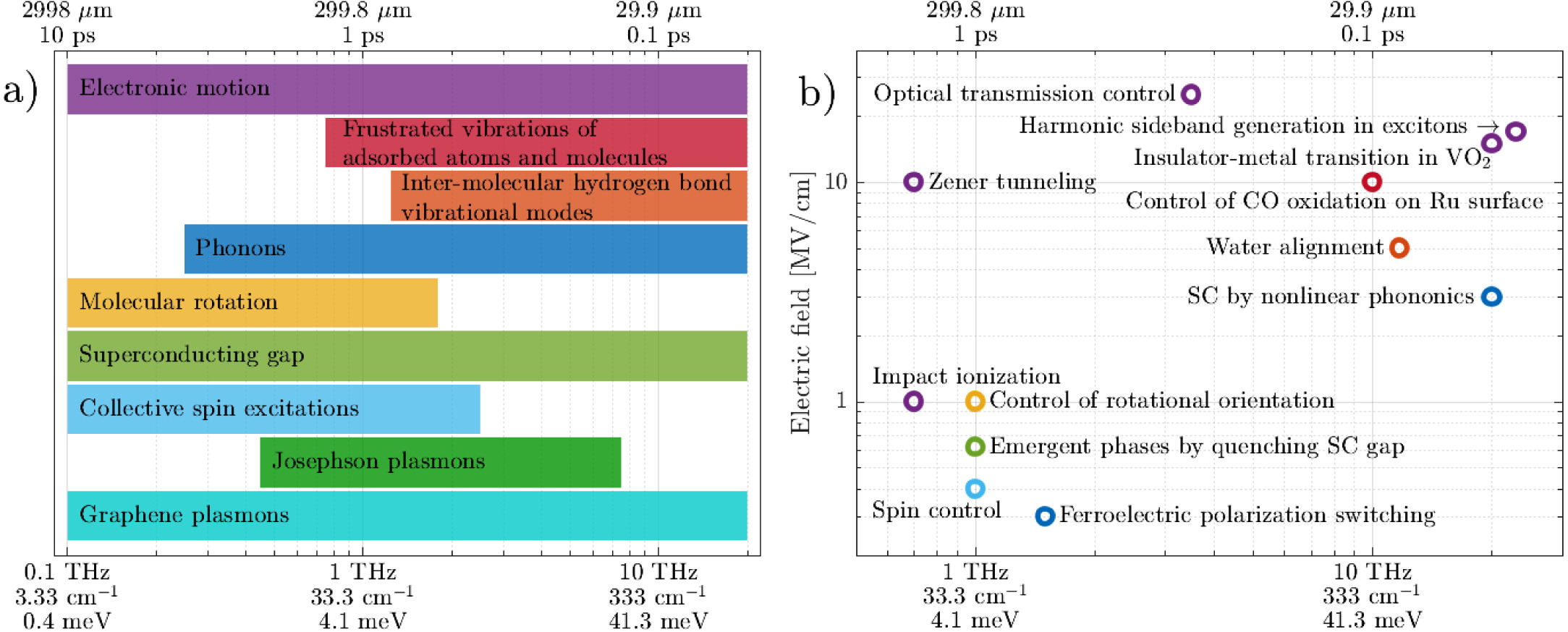
Now, a team of researchers has reviewed 1 around 200 studies on the interaction of strong-field THz pulses with various materials. Most of the reviewed studies have been performed during the last 5 years. Clearly, the field is very dynamic making past reviews obsolete.
The aim of this new review is threefold: first, to survey recent studies dealing with the transformation, control and engineering of the properties of various materials with strong-field few-cycle THz light; then, to introduce the operation principles for sources of strong-field few-cycle THz light and to survey the state-of-the-art performance of such sources; and, finally, to outline techniques for guiding, diagnosing and recomposing THz pulses.
Looking into the future, it is clear for the authors that further development of THz light sources that close the Thz gap will have a strong impact by providing the tool that enables presently unfeasible experiments. A highly beneficial feature of novel THz light sources will be the ability to reach the 5–15 THz spectral region. In this frequency range, present laser-based THz sources, relying on optical rectification, normally do not operate because of absorption in crystals, while the broad bandwidth of THz pulses generated in plasma results in a limited field amplitude per frequency unit.
On the other end, the authors conclude, even though the technology of THz detection is well established for strong-field (high-power) THz radiation, the development of high-sensitivity THz detectors will be beneficial for studies of nonlinear THz effects characterized by weak intensities. Graphene can be mentioned as one of several very promising materials for new THz detectors.
Author: César Tomé López is a science writer and the editor of Mapping Ignorance
Disclaimer: Parts of this article are copied verbatim or almost verbatim from the referenced research paper.
References
- PeterSalén, MartinaBasini, StefanoBonetti, JánosHebling, Mikhail Krasilnikov, Alexey Y. Nikitin, Georgii Shamuilov, Zoltán Tibai, Vitali Zhaunerchyk, Vitaliy Goryashko (2019) Matter manipulation with extreme terahertz light: Progress in the enabling THz technology Physics Reports doi: 10.1016/j.physrep.2019.09.002 ↩