The limits of Hyperloop
Author: Iván Rivera is an engineer working in Spanish and European R&D projects, conceiving and bringing to market innovative products and services for the railway sector. He is currently Innovation Manager at TELICE , S.A.
During 2017 we have been witnessing the first tests of what might be called an integral hyperloop system at the Virgin Hyperloop One installations in Las Vegas, Nevada. Virgin Hyperloop One — named Hyperloop One before Virgin Group’s entry into its shareholder structure — leads, by its own merits, the pack of companies pursuing the goal of building a practical hyperloop. It’s hard by now to be oblivious of the proposal for a so-called fifth transport mode developed by rocket and electric vehicle tycoon Elon Musk, of SpaceX and Tesla fame. Any and all hyperloop-related announcements, tests or infrastructure proposals from the Hyperloop Alpha 2013 white paper [1] onwards seem to have a huge impact on media.
In short, hyperloop is a system of transport capsules moving through nearly airless tubes without wall contact. The capsules accelerate and brake by means of linear induction motors located at regular intervals within the internal surface of the tube. Such a proposal can surprise by the boldness of its approach to high-speed travel, but even a cursory reading of technical specifications and cost estimations proposed by Musk takes that boldness into farfetchedness terrain in the minds and articles of transport experts [2][3]. Furthermore, the own progress of Virgin Hyperloop One’s physical implementation of the hyperloop concept show, beyond theoretical analyses, which problems shall have to be tackled in the near future, not without the potential of bringing the whole efforts to a relatively early end.
During the wee hours of Saturday, the 29th of July, Virgin Hyperloop One finished its first “Phase 2” test [4]. The testing capsule, by the name XP-1, covered 437 meters inside a tube where air pressure was slightly below 100 Pa (a thousandth of an atmosphere), reaching in the process a maximum speed of 310 km/h. To accomplish it, a linear induction motor with a 300 meters stator was used. With those starting data and some elementary kinematics, several parameters of the test can readily be deduced.
Assuming an initial resting state for the capsule and constant acceleration, we can compute that the value of acceleration should have reached 12.36 m/s² — 1.26 times the acceleration of gravity. Braking was somewhat more intense, reaching up to –2,76 g. Fortunately, XP-1 is no more than a chassis covered by a hollow fiberglass structure [5]. Potential passengers might have found the short trip extremely uncomfortable. It should be kept in mind, however, that Virgin Hyperloop One’s test track length is quite limited in length, imposing hard accelerations and decelerations if the system is to be tested at any significative speed.
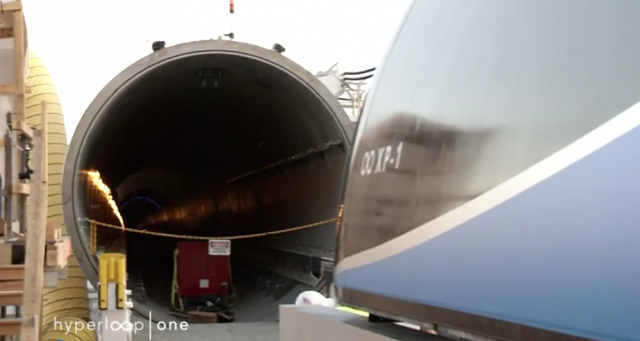
Virgin Hyperloop One’s tests are scantily documented. However, some analysis of published videos and other press materials should enable some inferences beyond simple kinematics. First of all, it is important to ascertain approximate capsule dimensions. The available documentation quotes 8,69 meters for length and 2,71 meters for height. However, the width, a fundamental parameter to calculate its cross-section, is nowhere to be found. We might conjecture, using video images, that the inner diameter of the tube ought to be 4 meters wide, while the capsule itself should be around three meters wide.
Correcting for the non-rectangular shape of the capsule’s cross-section we could infer an approximate surface of 6 m², while the tube itself would have an interior cross-section of 12.67 m². These values are consistent with the stated intention of Virgin Hyperloop One to zero in on a freight hyperloop, different to the first of the designs proposed by Musk in his white paper, but similar to the second one, although he conceived it originally as a mixed freight and passenger system, able to carry vehicles like a Tesla S — of course. All things considered and keeping in mind the uncertainty of estimated measures, we can state that the capsule takes around half of the available tube cross-section. Why is this important?
The Kantrowitz Limit
In the white paper which originated the hyperloop concept, Musk drew attention over a key issue in gas dynamics that acts as a relevant limit condition for the design of a transport system which must work inside of a tube in a transonic — close to sound speed — regime, by analogy to the airflow through a jet engine air inlet: the Kantrowitz limit. Arthur Kantrowitz, an American engineer, derived the expression for the stalling condition of a gas flow at transonic speeds forced to pass through a narrower path [6]. As the gas flow cannot be discontinuous, a constriction in its path forces a corresponding increase in its speed. When gas speed reaches the local sound velocity, the flow stalls.
In a hyperloop system, the Kantrowitz limit can be thought of as a “syringe effect”. Consider an empty syringe with its plunger fully pulled out: if the nozzle is blocked stopping any potential airflow, the plunger cannot be pushed forward without compressing all the air in front of it. The resistance to the plunger’s advance is in this case much greater than the one which would appear with an unblocked nozzle. Kantrowitz limit in hyperloop appears when the air, forced to pass through the free space between inner tube walls and capsule, reaches a critical speed beyond which flow stalls and the capsule starts behaving like a piston. A drag force originating from the pressure differential in front of and behind the capsule appears and increases exponentially with advance, independently of the initial air pressure — which does not even appear in the limit expression [7].
The estimates previously considered over the XP-1 capsule dimensions allow computing a possible Kantrowitz limit speed for the Virgin Hyperloop One test rig: approximately Mach 0.55 (188 m/s² or 677 km/h, assuming an inner tube temperature of 20 °C). That is a somewhat dismal record, considering that the official Virgin Hyperloop One account of the test contains the following quote:
With an additional 2000 meters of stator, we would have hit 1100 km per hour […]
Quite boastful but also likely untrue in light of the physical limits previously shown — unless the drive system can deliver enough power to overcome the increased drag force due to air pressures doubling each time the capsule halves the distance towards the tube’s end. However, this would disrupt energy consumption estimations. The “Phase 3” test carried out on December 15, 2017 [8], while reaching a new speed record of 387 km/h, also took place at an inner tube pressure of 25 Pa, which is already four times less than envisaged by Musk. Is Kantrowitz limit approaching already for Virgin Hyperloop One engineers? We should keep in mind that hyperloop is a delicate balancing act — and in particular, lowering pressures will increase final system costs, likely in a non-linear way.
Musk’s original white paper correctly discussed the relevance of the Kantrowitz limit for hyperloop-like systems. Speeds envisaged by both Musk and Virgin Hyperloop One for the finished system are around Mach 0.9 (1100 km/h); using Kantrowitz’s formula, the ratio of free to total cross-section for such a speed is 0.99. That is, a hyperloop could only work below Kantrowitz limit inside an enormously wide tube relative to capsule’s cross-section, thus eliminating any possible advantage that the tube presence could entail. But Musk addressed this issue in quite an ingenious way: why not fit the capsule front with an axial compressor, similar to the one found in turbojets? In this manner the capsule itself would move the air in front to its back, increasing the free cross-section as far as the compressor efficiency could allow. Going a step further, Musk and his team figured that the compressor ought to work at a 20-to-1 compression ratio. They too performed the necessary calculations to harness part of the air to cover for life support systems needs on board the capsule. At the same time, the temperature increase of compressed gases in adiabatic conditions was taken into account.
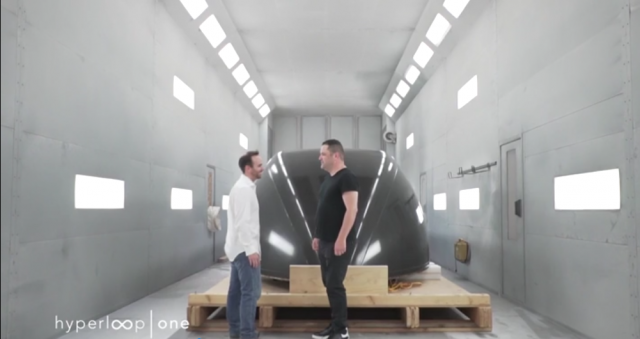
All these considerations notwithstanding, a simple visual inspection of the XP-1 capsule rules out the presence of a front axial compressor — something, by the way, featured in nearly all artist recreations of hyperloop capsules, including the one heading this text, created by Virgin Hyperloop One (though no longer available in their website). This lack of a frontal compressor is the defining characteristic that allows such a confident estimation of the speed at which the XP-1 capsule will reach the Kantrowitz limit. But would not simply installing a compressor be enough to do away with the problem? After all, Dirk Ahlborn, CEO of Hyperloop Transportation Technologies — and a direct competitor of Virgin Hyperloop One for the crown of the budding hyperloop market — said early in 2017 that “the technology [to build a hyperloop] is not the issue – it already exists” [9].
This claim is false. At present, there is no axial compressor on the market with the features needed to equip a hyperloop capsule like the XP-1 — or any other full-scale design, for that matter. Air compressors used in aviation jet engines go routinely over 20-to-1 compression ratios but need kerosene to work. Moreover, no jet-propelled aircraft has ever flown over the 37.65 kilometers record set by A. V. Fedotov in a MiG-25M on 1977 — and the atmospheric pressure at that altitude is still four times greater [10]. It is no doubt possible to develop an electric axial compressor with a 20:1 ratio, although one could be excused to doubt about its ability to operate in such a low-pressure regime. A simple fact like this should compel would-be hyperloop sellers to be much more prudent regarding availability timescales and abstain themselves from issuing the usual readiness statements; this also holds for uncritical media outlets. Finally, the electric consumption of this wholly theoretical compressor is a great unknown, even though simple thermodynamic calculations should allow establishing a lower bound.
For this reason alone, the energy budget of the system as per Musk’s white paper ought to be sharply questioned. In addition, if we consider that energy consumption shown for railways among other transport modes in its first figure (shown below) was clearly — perhaps intentionally — wrong, it is by no means senseless to challenge the viability of the hyperloop system as a whole.
To have or have not (a compressor)?
At this stage, we find a dilemma: either the electric axial compressor with the needed features and performance is developed, or it is skipped. In the first case the capsule speed could theoretically be brought up to a near-transonic regime; then the system would encounter a thermodynamic hurdle which the original white paper tiptoes around. Any gas subject to a fast compression will increase its temperature. Therefore, something must be done about this additional energy if we do not expect the capsule to act as a hot air oven — such a device may be exactly what is needed to cook crispy fries without using much oil, but is less than ideal when talking about moving around people or freight. Musk conceives of a water-based cooling subsystem, a pair of intercoolers and several tanks to store water and overheated steam. The carrying capacity of these tanks is readily calculated and then, in a single line of terse description (in section 4.1.3), done away with:
Water and steam tanks are changed automatically at each stop.
This interesting, non-trivial and underreported step brings to mind a certain reminiscence of steam engines of yore. It also has the undesired effect of complicating capsule management logistics; something that in order to maintain the foreseen passenger flow of the system ought to be carried out with the utmost precision and swiftness, lest the passenger per hour metric (and thus profitability) be harmed beyond repair. Furthermore, its an added encumbrance to the foreseen automatic change of batteries for capsules at each trip’s end: as capsules cannot obtain energy from infrastructure as an electric train would do, all internal operations (including compressor, intercoolers, valves and life support systems, among others) has to be battery-based.
Musk himself, through his automotive company Tesla, has advocated for years the creation of a fast battery switching infrastructure for his electric cars. This would have the obvious advantage of superseding potentially very long recharging times for car batteries with a futuristic and automated pass through a robotic battery swap station. Unfortunately, the growing availability of fast charging points — and, potentially, the introduction of a key failure point in the main connection for an electric vehicle, with the correspondingly foreseeable reduced reliability — have precluded this technology to develop [12]. More so, there are no prototypes that could be fitted into a hyperloop capsule; much less when the pieces of equipment being exchanged are not just batteries with electric contact, but compressed air and overheated steam tanks that must be secured to the ensemble with sturdy valves.
Let us then return to the second possibility in our compressor dilemma: to forgo its use and operate the system at subsonic speeds below Mach 0.5 to keep energy use as low as envisaged. A hyperloop running at a peak speed around 600 km/h might well be buildable, but it is considerably less clear whether the resulting mass transport could be attractive in terms of construction costs per kilometer, maintenance costs, energy consumption and comfort compared to its more obvious competitor, the high-speed train or its purported successor, already decades in the making by companies and public entities alike in the U.S.A., Germany, Japan and China: the magnetic levitation train or maglev.
There is just one high-speed maglev line in the world in commercial operation: the Shanghai Transrapid, covering a distance of 30.5 kilometers in eight minutes at a peak record speed of 430 km/h. All other maglevs in existence are in slow or medium speed lines built around the people mover concept frequently seen in main airports. Japan is now building the Chūō Shinkansen line [13][14], a very ambitious undertaking with a similar profile to the one aspired to by hyperloop advocates: it will cover the journey from Tokyo to Nagoya and Osaka in a little over an hour, reaching speeds up to 505 km/h. Current plans for this engineering marvel — with more than 86% of the Tokyo-Nagoya stretch executed underground — foresee commissioning of its first section in 2027, with the Osaka arrival planned for 2045. These are realistic deadlines for cutting-edge infrastructure and not those ludicrous four years insisted upon by those in hyperloop entourage.
Even with serious engineering plans laid out, most mass transit experts tend to support the view that huge maglev projects are nothing but “white elephants” [15]: high visibility works whose intent is more to emphasize a country’s technical prowess than to attain a new and practical way to move lots of people between populous destinations. Potential speed increases in a maglev system entail correspondingly elevated building costs per kilometer, which are only justified with a very pronounced transport demand. In the absence of such conditions and sporting a non-existent technological risk, high-speed rail is the alternative to consider. Reliable [16] and mature, but still with some margin for improvements, high-speed rail can connect cities separated by hundreds of kilometers with known costs and minimum environmental impact.
The hyperloop concept raises other, no less important doubts and potential limitations beyond those considered here from technology, operation, and safety viewpoints. All things considered, it can be stated categorically that a passenger hyperloop will not come to pass — not in four years, as Virgin Hyperloop One boasts, not in twenty (a reasonable minimum for an engineering project with so much undefined technology, and perhaps never.
—
This article has been translated into English, reviewed and updated from the original “Los límites del Hyperloop”, published August 18, 2017, in Cuaderno de Cultura Científica (https://culturacientifica.com/2017/08/18/los-limites-del-hyperloop/) by its author, Iván Rivera.
References:
[1] Musk, E. R. et al. (2013, August 12). Hyperloop Alpha. Retrieved August 14, 2017, from http://www.spacex.com/sites/spacex/files/hyperloop_alpha-20130812.pdf.
[2] Bradley, R. (2016, May 10). The Unbelievable Reality of the Impossible Hyperloop, MIT Technology Review. Retrieved August 14, 2017, from https://www.technologyreview.com/s/601417/the-unbelievable-reality-of-the-impossible-hyperloop/.
[3] Konrad, A. (2016, November 11). Leaked Hyperloop One Docs Reveal The Startup Thirsty For Cash As Costs Will Stretch Into Billions, Forbes. Retrieved August 14, 2017, from https://www.forbes.com/sites/alexkonrad/2016/10/25/hyperloop-one-seeks-new-cash-amid-high-costs/#4f78547d125c.
[4] Giegel, J., Pishevar, S. (2017, August 02). Hyperloop Gets More Real Every Time We Test, Hyperloop One. Retrieved August 14, 2017, from https://hyperloop-one.com/blog/hyperloop-gets-more-real-every-time-we-test.
[5] Hyperloop One. (2017, August 10). What Testing Has Taught Us. Retrieved August 14, 2017, from https://hyperloop-one.com/blog/what-testing-has-taught-us.
[6] Kantrowitz, A., Coleman, duP. (1945, May). Preliminary Investigation of Supersonic Diffusers. Advance Confidential Report L5D20, National Advisory Committee for Aeronautics. Retrieved August 14, 2017, from http://www.dtic.mil/dtic/tr/fulltext/u2/b184216.pdf.
[7] An appropriate expression for the Kantrowitz limit as applicable to hyperloop is:
Where Abypass is the free cross-section, Atube the total inner cross-section, γ the isentropic expansion coefficient and M the Mach number of the airflow (equivalent to capsule speed).
[8] Giegel, J., & Lloyd, R. (2017, December 18). New Chairman, New Funding, & New Speed Records. Retrieved December 30, 2017, from https://hyperloop-one.com/blog/new-chairman-new-funding-new-speed-records.
[9] Carlström, V. (2017, January 26). HTT’s CEO says a hyperloop line will be up and running within three years: “The technology is not the issue – it already exists”, Business Insider Nordic. Retrieved August 14, 2017, from http://nordic.businessinsider.com/htts-ceo-says-a-hyperloop-line-will-be-up-and-running-within-three-years-the-technology-is-not-the-issue–it-already-exists-2017-1/.
[9b] Glenn Research Center, NASA. Earth Atmosphere Model. Retrieved December 29, 2017, from https://www.grc.nasa.gov/www/k-12/airplane/atmosmet.html.
[10] García Álvarez, A. (2008, January). Consumo de energía y emisiones del tren de alta velocidad en comparación con otros modos. Vía Libre, (515) (in Spanish). Retrieved August 14, 2017, from https://www.vialibre-ffe.com/PDF/Comparacion_consumo_AV_otros_modos_VE_1_08.pdf.
[11] Korosec, K. (2015, June 10). Tesla’s battery swap program is pretty much dead, Fortune. Retrieved August 14, 2017, from http://fortune.com/2015/06/10/teslas-battery-swap-is-dead/.
[12] Chuo Shinkansen Maglev Line. Railway Technology. Retrieved August 14, 2017, from http://www.railway-technology.com/projects/chuo-shinkansen-maglev-line/.
[13] JR Central (2012). The Chuo Shinkansen using the Superconducting Maglev System. JR Central Annual Report. Retrieved August 14, 2017, from http://english.jr-central.co.jp/company/ir/annualreport/_pdf/annualreport2012-05.pdf.
[14] Binning, D. (2008, February 11). Maglev – the Great Debate, Railway Technology. Retrieved August 14, 2017, from http://www.railway-technology.com/features/feature1606/.
[15] European Commision. (2017, February 10). Rail accident fatalities in the EU. Retrieved August 14, 2017, from http://ec.europa.eu/eurostat/statistics-explained/index.php/Rail_accident_fatalities_in_the_EU.
5 comments
[…] ne peut dépasser une certaine vitesse du fait de l’air qui se comprime devant lui. D’après The limits of Hyperloop publié par Ivan Rivera en février 2018, le véhicule de test d’Hyperloop One dit XP1 ne […]
[…] Hyperloop, & Musk, E. (2018, February 07). The limits of Hyperloop. Retrieved from https://mappi… […]
[…] Rivera Rodríguez, I. (07/02/2018). The limits of Hyperloop. Mapping Ignorance. Visitado el 13/08/2022 en https://mappingignorance.org/2018/02/07/the-limits-of-hyperloop/. […]
[…] Rivera Rodríguez, I. (07/02/2018). The limits of Hyperloop. Mapping Ignorance. Visitado el 13/08/2022 en https://mappingignorance.org/2018/02/07/the-limits-of-hyperloop/. […]
[…] ese prestigiosa web de divulgación científica en inglés. De modo que, sin más preámbulo… «The limits of Hyperloop» en Mapping […]