Towards advanced room-temperature valleytronic nanodevices.
So-called “valleytronics” is a new type of electronics that could lead to faster and more efficient computer logic systems and data storage chips in next-generation devices. Valley electrons are so named because they carry a valley degree of freedom, a pseudospin. This is a new way to harness electrons for information processing that’s in addition to utilizing an electron’s other degrees of freedom, which are quantum spin in spintronic devices and charge in conventional electronics.
The optical properties of two-dimensional (2D) semiconductors are dominated by excitonic effects. In particular, two-dimensional transition metal dichalcogenides (TMDCs) have a direct bandgap in the visible region at the energetically degenerate K and K′ (−K) points of their hexagonal Brillouin zones, the so-called valleys. In other words, two electrons can have the same energy but different wavelengths – or, to put it another way, different valley.
TMDCs can therefore support two different types of energetically degenerate exciton, which are identical except that they have opposite Berry curvatures. The interband transitions in the vicinity of the K (or K’) point are coupled to right (or left) circular photon polarization states. Consequently, the two types of exciton exhibit distinct responses to light of different helicity depending on their valley pseudospin.
These valley dependent optical selection rules arise from inversion symmetry breaking. This provides a convenient method to address specific valley states (K+ or K-) by circularly polarized (right or left) optical excitation. To take advantage of this effect and build feasible valleytronic devices for optoelectronic applications, this valley degree of freedom must be accessed through the different responses to external stimuli such as electric, magnetic and optical fields.
Even though some applications in optoelectronics and valleytronics have been realized using the spin–valley effect, a problem persists: the lifetime of the valley excited state is typically very short, especially at room temperature, making it difficult to maintain the coherence of the valley and manipulate its index. Moreover, light–matter interaction in atomically thin TMDCs is very weak, and even weaker in nonlinear conversion processes, as is the case when two photons with the same frequency interact with a nonlinear material, are “combined”, and generate a new photon with twice the energy of the initial photons, what is called second-harmonic generation.
These limitations hinder the possibility of nonlinear valleytronic devices based on 2D TMDCs, which are necessary for valley-multiplexed data transport15–19. Therefore, it is important to establish viable approaches to the design of nanophotonic structures that, when combined with 2D TMDCs, could both boost the efficiency of nonlinear processes and exploit their valley index.
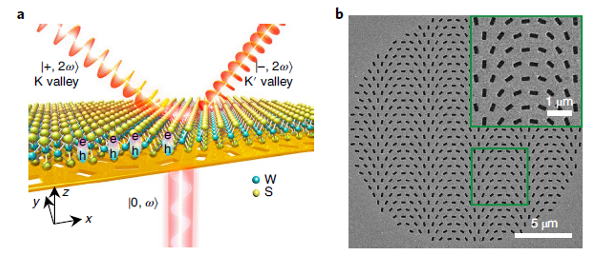
Now, an international team of researchers, including Francisco García-Vidal (IFIMAC-UAM & DIPC), theoretically propose and experimentally demonstrate 1 a metasurface-assisted photonic pathway to boost the coherent spin–valley-dependent nonlinear optical process in monolayer TMDCs and to steer the nonlinear photons from different valleys in any desired direction in free space at room temperature.
An electromagnetic metasurface is any kind of artificial sheet material with sub-wavelength thickness. Optical metasurfaces have emerged as important flat-profile platforms in nanophotonics capable of manipulating light properties including phase, amplitude, spin, frequency and chirality. In particular, plasmonic metasurfaces in which a photonic spin–orbit interaction is devised can discriminate photon spins and apply a spin-related geometric phase to light. In this way, different spin components can be spatially separated, an effect known as the photonic spin-Hall effect.
The new synthetic Au–WS2 metasurface contains two parts: the plasmonic Au metasurface and the monolayer of the TMDC WS2 on top of it. This metasurface is designed in such a way that the spin–valley-exciton-locked second-harmonic generation occurs at the excitonic resonance of the K and K’ valleys in monolayer WS2 .
The plasmonic metasurface is able to enhance the nonlinear process responsible for the second-harmonic generation due to the large plasmonic field localization around the nanoholes. The Au metasurface imposes a chirality-dependent phase gradient, which arises from the photonic spin–orbit interaction, to the fundamental-frequency light. This gradient implies an enhancement of the second-harmonic generation by one order of magnitude and, consequently, steers nonlinear chiral photons emitted from different valleys in different spatial directions.
These results could provide a way to manipulate the valley-exciton transport, steer the spin–valley-exciton emission with nonlinear chiral valley–photon interfaces, and encode the valley index into free space or, simply put, may facilitate advanced room-temperature and free-space nonlinear, quantum and valleytronic nanodevices.
Author: César Tomé López is a science writer and the editor of Mapping Ignorance
References
- Guangwei Hu, Xuanmiao Hong, Kai Wang, Jing Wu, He-Xiu Xu, Wenchao Zhao, Weiwei Liu, Shuang Zhang, Francisco Garcia-Vidal, Bing Wang, Peixiang Lu & Cheng-Wei Qiu (2019) Coherent steering of nonlinear chiral valley photons with a synthetic Au–WS2 metasurface Nature Photonics doi: 10.1038/s41566-019-0399-1 ↩