High temperatures and strong random interactions need not destroy many-body quantum entanglement
One of the most mysterious features of quantum mechanics is that if two particles (or photons) interact at some point in time then the properties of these particles will remain connected at future times. A consequence of this is that determining the quantum state of one of the particles simultaneously determines the quantum state of the other, even if the two particles are very far apart.
That quantum mechanics leads to this conclusion was first pointed out by Albert Einstein, Boris Podolsky and Nathan Rosen in 1935. This connectedness between particles was called entanglement by Erwin Schrödinger in a paper also published in 1935 in which he emphasized that it is a key feature of quantum mechanics that distinguishes it from classical physics.
For many years quantum entanglement was a purely theoretical topic of interest to people concerned with the philosophical foundations of quantum mechanics. This situation changed dramatically in 1964 when John Bell showed theoretically that experimental tests could be carried out to see whether the idea of quantum entanglement was correct. The first experiments to investigate quantum entanglement were performed by Alain Aspect and his colleagues in the 1980s. They showed that quantum entanglement is a real phenomenon.
It is possible to extend quantum entanglement to three or more particles. It was shown by Daniel Greenberger, Michael Horne and Anton Zeillinger in 1987 that, whereas in two-particle entanglement the differences between local realism and quantum mechanics are statistical, with three or more particles the differences can sometimes be stated as certainties.
Decoherence, on the other hand, is the enemy of entanglement. It is a process in which a quantum mechanical state of a system, like entanglement, is altered by the interaction between the system and its environment. Quantum technologies use entanglement to outperform classical technologies, and often employ strong cooling and isolation to protect entangled entities from decoherence by random interactions – the entropy of the environment.
But, what if the contrary were true? Could it be possible that promoting random interactions can help generate and preserve entanglement? If true, this could be most important for many applications of quantum entanglement like quantum computation, simulation, and sensing, but it would be also relevant from a fundamental point of view, as it is believed to underlie important many-body phenomena such as high temperature superconductivity.
Now, a team of researchers has showed experimentally 1 that it is possible.
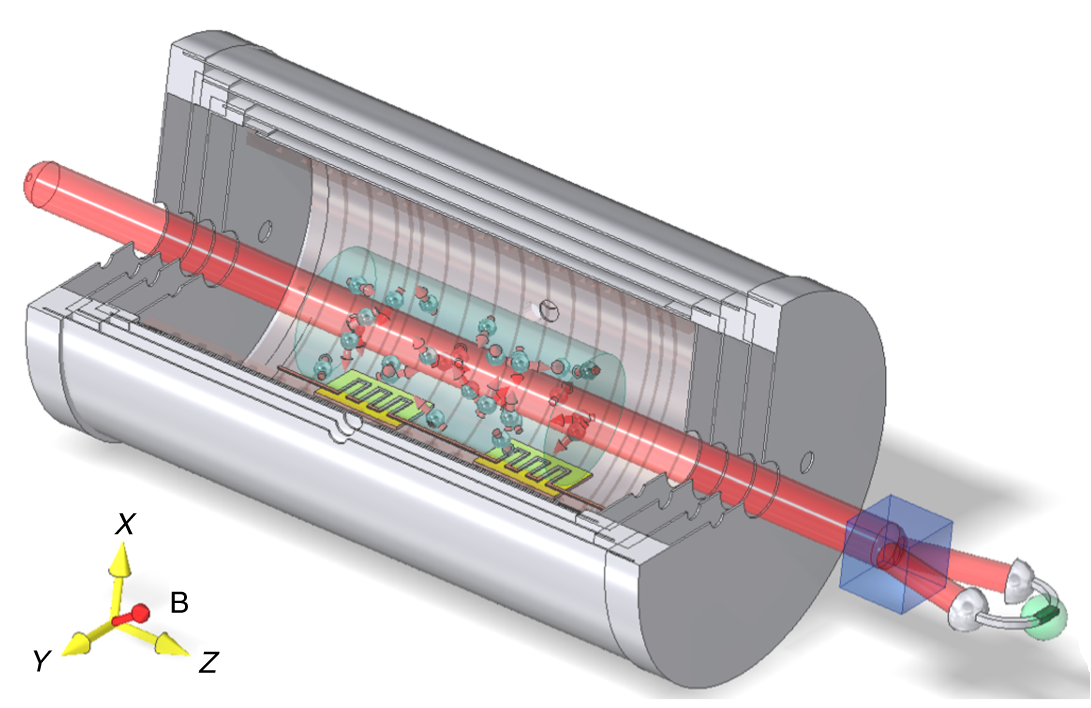
On the one hand we find that, as expected, in many quantum technological implementations, strong cooling and precise controls are required to prevent entropy—whether from the environment or from noise in classical parameters—from destroying quantum coherence. Quantum sensing is often pursued using low-entropy methods, for example, with cold atoms in optical lattices. But, on the other hand, there are important sensing technologies that operate in a high-entropy environment, and indeed that employ thermalization to boost coherence and thus sensor performance. The question is then whether entanglement can be generated, survive, and, importantly, be observed in a high entropy environment.
The interaction of quantum spins with their environment introduces relaxation and decoherence in the otherwise fully coherent evolution of ideal closed quantum systems. Spin relaxation and decoherence play a central role in many branches of physics. In the case of nuclear spins, the time scales associated to energy relaxation and decoherence provide a very meaningful information of the environment that forms the basis of magnetic resonance imaging techniques. The spin relaxation and decoherence time scales set the limit of sensitivity in several existing magnetometry techniques, such as optically detected magnetic resonance and spin-exchange relaxation-free (SERF) atomic magnetometry, and are also one of the major constraints in the implementation of spin-based quantum computers, such as donors in silicon, electrons in quantum dots and even molecular magnets.
In the SERF regime, strong, frequent, and randomly-timed spin-exchange collisions dominate the spin dynamics, to produce local spin thermalization. The researchers have now studied the nature of spin entanglement in this hot, strongly-interacting atomic medium, using techniques of direct relevance to extreme sensing. They applied optical quantum non-demolition measurement—a proven technique for both generation and detection of non-classical states in atomic media—to a SERF-regime vapor. The experimental set-up was a vapor of 87Rb atoms contained in a glass cell with buffer gas to slow diffusion, and housed in magnetic shielding and field coils to control the magnetic environment.
The use of Bayesian statistics and spin-squeezing inequalities let the scientists show that at least a 28 % (1.52 × 1013 out of 5.32 × 1013) of the participating atoms enter into singlet-type entangled states, which persist for tens of spin-thermalization times and span thousands of times the nearest-neighbor distance.
This is a concrete and experimentally tractable example of a system in which entanglement is not only compatible with, but, in fact, stabilized by entropy-generating mechanisms—in this case strong, randomly-timed spin-exchange collisions.
It is particularly intriguing that the observed macroscopic singlet state shares several traits with a spin liquid state, which is conjectured to underlie high-temperature superconductivity, a prime example of quantum coherence surviving in an entropic environment.
The results show that high temperatures and strong random interactions need not destroy many-body quantum coherence, that collective measurement can produce very complex entangled states, and that the hot, strongly-interacting media now in use for extreme atomic sensing are well suited for sensing beyond the standard quantum limit.
Author: César Tomé López is a science writer and the editor of Mapping Ignorance
Disclaimer: Parts of this article may be copied verbatim or almost verbatim from the referenced research paper.
References
- Kong, J., Jiménez-Martínez, R., Troullinou, C. Vito, Giovanni Lucivero, Géza Tóth & Morgan W. Mitchell (2020) Measurement-induced, spatially-extended entanglement in a hot, strongly-interacting atomic system. Nature Communications doi: 10.1038/s41467-020-15899-1 ↩