Electroactive microenvironments as a major driver of cell behavior
“There is something at work in my soul, which I do not understand.”
― Mary Wollstonecraft Shelley (1797-1851), Frankenstein or The Modern Prometheus, 1818
Increasing advances in material’s science have enabled the design and production of materials capable of inducing specific cell behaviors, such as proliferation, migration or differentiation. Consequently, we are now able to develop new implants that mimic the natural properties of tissues in order to guide the regeneration and repair of damaged tissues. Cell microenvironment is a complex niche in which biochemical and biophysical cues play a decisive role in determining cell fate (Razafiarision et al., 2016). Among the properties known to affect cell fate, mechanical and electrical signals are ubiquitous in cell microenvironment and function, and must be tailored for the development of advanced tissue regeneration strategies (Khare et al., 2020). In particular, electromechanical signals are essential components of the musculoskeletal system, heart and skin, among other tissues. To suitably control and provide those signals during tissue regeneration, advanced and multifunctional materials are increasingly being required with tailored active physical-chemical properties and time response (Ribeiro et al., 2015a). In this context, piezoelectricity appears as a promising and needed strategy for the next generation of advanced materials for biomedical applications.
Piezoelectricity refers to the intrinsic property of dielectric materials to generate an electrical potential when subjected to mechanical deformation, usually called the “direct piezoelectric effect”. In piezoelectric materials, the relationship between the applied mechanical stress and the induced electrical response is linear and reversible. Consequently, after implantation, piezoelectric materials can deliver electrical cues without the requirement of an external stimulation device as their electric activity is induced by physiological mechanical deformations.
As previously stated, electrical cues, and in particular electromechanical signals, constitute one of the main physical stimuli present in the human body, with tissues of the musculoskeletal system, such as bone and tendon, being particularly electro-mechanically active. Consequently, a number of piezoelectric materials, including polymers, ceramics and composites, have been explored as bioactive scaffolds to stimulate bone regeneration. Further, biomaterials such as cellulose, poly-L-lactic acid, some amino acids, peptides and proteins, including collagen, also show piezoelectric response.
While different piezoelectric materials are being tailored for their use in biomedical applications, poly(vinylidene fluoride) (PVDF) and its copolymers have been the most explored materials as they display low cytotoxicity, high piezoelectric coefficients, and can be easily processed into different conformations, such as fibers, spheres, films and three-dimensional scaffolds (Ribeiro et al., 2018a). Semi-crystalline PVDF can show up to five different polymorphs (namely, α, β, γ, δ, ε) depending on the processing conditions. To obtain PVDF with optimized piezoelectric effect, methods such as annealing, stretching and application of electric fields during polymerization are typically used to produce PVDF with high β-phase content, which has the highest piezoelectric coefficient. In turn, the PVDF copolymer poly[(vinylidenefluoride-co-trifluoroethylene] (PVDF-TrFE) displays high piezoelectricity and electromechanical coupling coefficient without the need of applying mechanical strain or electric fields during its preparation. As we will see, piezoelectric PVDF and PVDF-TrFE have been widely used to prove the relevance of dynamic electric stimulation for the regeneration of musculoskeletal tissues.
Preosteoblastic cells have been reported to spread to a greater extent and proliferate more rapidly when adhered to positively poled PVDF surfaces. However, mesenchymal stem cells (MSCs), which are multipotent cells capable of differentiating into cells of the bone lineage, show increased formation of focal adhesions when grown on negatively poled substrates compared to the neutral and positively charged ones. When mechanical stress is applied, triggering the piezoelectric effect, MSCs show increased differentiation on both, positively and negatively poled PVDF surfaces, compared to the neutral ones, demonstrating the importance of combined electrical and mechanical stimulation for bone regeneration (Sobreiro-Almeida et al., 2017; Ribeiro et al., 2015b). In contrast to preosteoblastic cells, skeletal muscle cells proliferate faster on negatively poled PVDF substrates. Nevertheless, regardless of the polarity state of the material, the morphology adopted by muscle cells growing on flat surfaces does not resemble that of mature cells, indicating the need for geometric cues to better simulate their mechanoactive niche. Given the ease of processing PVDF and the ability to generate fibers and three-dimensional scaffolds in the poled state, it represents a unique material to analyze the combined effect of topographic and electrical influences on the above processes. Indeed, more physiological myoblast morphologies are achieved by using electrospun PVDF fibers on which cell differentiation is simulated while muscle cells adopt aligned morphologies (Ribeiro et al., 2018b, 2020a & 2020b). Similarly, the effect of topography seems to play a role in the osteogenic process. When comparing the osteogenic potential of preosteoblastic cells attached to PVDF-TrFE with patterned hexagons and lines, it was shown that the isotropic hexagonal surface topography could promote osteogenesis without the addition of chemical factors (Marques-Almeida et al., 2020).
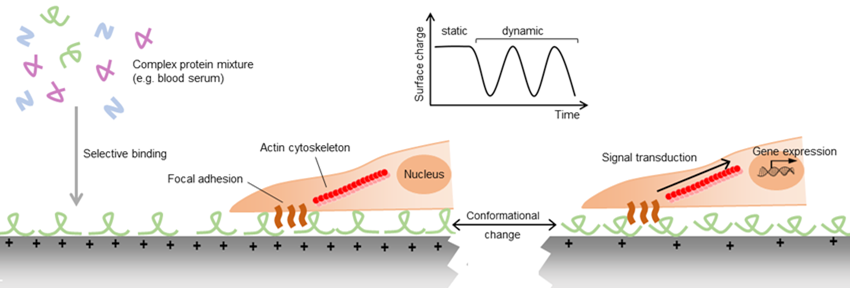
Despite the abundant evidence for the importance of piezoelectric stimulation on cell behavior, the precise mechanisms controlling this response remain largely undescribed. It is known that the binding of proteins to biomaterials, either immobilized during the fabrication of the material or adsorbed from body fluids after implantation, is essential to the subsequent biological response to the material. For example, the wettability of soft materials has been shown to regulate the differentiation of mesenchymal stem cells (MSCs) into bone cells via a mechanism involving the exposure of cryptic adhesion sites of collagen molecules adsorbed to hydrophilic surfaces (Razafiarison et al., 2018). Similarly, surface charges can affect the orientation and conformation of the proteins adhered to them and consequently alter the interaction of cells with the protein coating (Ribeiro et al., 2012). In addition to these conformational differences, adsorption of proteins from complex protein mixtures is selective, potentially leading to different protein compositions, and consequently different cell binding motifs on materials of different surface electric charge. As in piezoelectric materials the net electric charge can be altered by the application of mechanical strain, these materials can potentially allow the dynamic modification of cell adhesion (Figure 1).
Taken together, it is clear that materials able to provide electromechanical cues and, in particular, piezoelectric polymers such as PVDF and PVDF-TrFE, hold great promise in the field of tissue engineering. Nevertheless, to design of a new generation of electromechanically active biopolymers with tailored response and morphology, deeper knowledge of the mechanisms of cell differentiation driven by electric charges and the active manipulation of the protein interlayer are needed to further explore the full potential of this strategy and to reach translation to actual therapies.
Acknowledgments
The authors acknowledge funding by Spanish State Research Agency (AEI) and the European Regional Development Fund (ERFD) through the project PID2019-106099RB-C43/AEI/10.13039/501100011033 and from the Basque Government Industry and Education Departments under the ELKARTEK and PIBA (PIBA-2018-06) programs, respectively.
References:
Khare D, Basu B, Dubey AK. (2020). Electrical stimulation and piezoelectric biomaterials for bone tissue engineering applications. Biomaterials. 258:120280.
Marques-Almeida T, Cardoso VF, Gama M, Lanceros-Mendez S, Ribeiro C. (2020). Patterned Piezoelectric Scaffolds for Osteogenic Differentiation. Int J Mol Sci. 21:8352.
Razafiarison T, Silván U, Meier D, Snedeker JG. (2016). Surface-Driven Collagen Self-Assembly Affects Early Osteogenic Stem Cell Signaling. Adv Healthc Mater. 5:1481-92.
Razafiarison T, Holenstein CN, Stauber T, Jovic M, Vertudes E, Loparic M, Kawecki M, Bernard L, Silvan U, Snedeker JG. (2018). Biomaterial surface energy-driven ligand assembly strongly regulates stem cell mechanosensitivity and fate on very soft substrates. Proc Natl Acad Sci U S A. 115:4631-4636.
Ribeiro C, Panadero JA, Sencadas V, Lanceros-Méndez S, Tamaño MN, Moratal D, Salmerón-Sánchez M, Gómez Ribelles JL. (2012). Fibronectin adsorption and cell response on electroactive poly(vinylidene fluoride) films. Biomed Mater.7:035004.
Ribeiro C, Sencadas V, Correia DM, Lanceros-Méndez S. (2015a). Piezoelectric polymers as biomaterials for tissue engineering applications. Colloids Surf B Biointerfaces. 136:46-55.
Ribeiro C, Pärssinen J, Sencadas V, Correia V, Miettinen S, Hytönen VP, Lanceros-Méndez S. (2015b). Dynamic piezoelectric stimulation enhances osteogenic differentiation of human adipose stem cells. J Biomed Mater Res A. 103:2172-5.
Ribeiro C, Costa CM, Correia DM, Nunes-Pereira J, Oliveira J, Martins P, Gonçalves R, Cardoso VF, Lanceros-Méndez S. (2018a). Electroactive poly(vinylidene fluoride)-based structures for advanced applications. Nat Protoc. 13:681-704.
Ribeiro S, Gomes AC, Etxebarria I, Lanceros-Méndez S, Ribeiro C. (2018b). Electroactive biomaterial surface engineering effects on muscle cells differentiation. Mater Sci Eng C Mater Biol Appl. 92:868-874.
Ribeiro S, Puckert C, Ribeiro C, Gomes AC, Higgins MJ, Lanceros-Méndez S. (2020a). Surface Charge-Mediated Cell-Surface Interaction on Piezoelectric Materials. ACS Appl Mater Interfaces. 12:191-199.
Ribeiro, S., Ribeiro, C., Carvalho, EO, Tubio CR, Castro N, Pereira N, Correia V, Gomes, AC, Lanceros-Méndez, S. (2020b). Magnetically Activated Electroactive Microenvironments for Skeletal Muscle Tissue Regeneration. ACS Applied Bio Materials. 3:4239–4252
Sobreiro-Almeida R, Tamaño-Machiavello MN, Carvalho EO, Cordón L, Doria S, Senent L, Correia DM, Ribeiro C, Lanceros-Méndez S, Sabater I Serra R, Gomez Ribelles JL, Sempere A. (2017). Human Mesenchymal Stem Cells Growth and Osteogenic Differentiation on Piezoelectric Poly(vinylidene fluoride) Microsphere Substrates. Int J Mol Sci. 18:2391.
Authors
Unai Silván1,2& Senentxu Lanceros-Méndez1,2
1BCMaterials, Basque Center for Materials, Applications and Nanostructures, UPV/EHU Science Park, 48940 Leioa, Spain
2IKERBASQUE, Basque Foundation for Science, 48013 Bilbao, Spain