Is the valorization of marine organic-waste into chitin environmentally sustainable?
Authors: Mireia Martín, Life Cycle Thinking Group, Department of Graphic Design and Engineering Projects. University of the Basque Country (UPV/EHU) and Erlantz Lizundia, Life Cycle Thinking Group and BCMaterials
One of the consequences of global population growth is an increase in food waste. This is a relevant issue not only in terms of the waste of valuable resources, but also in terms of the environmental impact associated with the disposal of products at the end of their useful life. In fact, the UNEP Food Waste Index 2021 estimates food waste represents nearly the 8-10% of global greenhouse gas emissions. Therefore, finding new ways to reduce food waste should be a priority for scientist, industry and policy makers (Roy et al., 2023).
Circular economy within the primary resource, waste management and CO2 emission crisis
It seems that the scientific interest is moving in this direction. Food Supply Chain Waste (FSCW), which includes agricultural and processing waste from food production chains, is seen as an exciting new area for upcycling materials and put them back into the economic cycle. So far, a large fraction of the research in this area has focused on the production of biofuels, fertilizers, animal feed and packaging materials. In spite of the important source of proteins, polysaccharides, minerals and lipids that can be isolated from food waste, a little attention has been focused on its valorization in the form of functional materials.
Valorization of co-products remains at the core of bioeconomy
Here we focus our attention on chitin, the second most abundant polysaccharide after cellulose (it is a linear polymer composed of repeating β(1,4)-N-acetylglucosamine units) that finds many applications in plethora of fields within the materials science and biotechnology. In particular, we consider its use for the production of chitin nanocrystals (ChNC) from food waste, a hot topic in food processing and recycling for technological applications. Chitin can be obtained from the exoskeletons of insects, crustaceans (mainly arthropods) and mollusks, and it could be found at the cell walls of fungi. It is an interesting biopolymer found in living organisms and meets current requirements for availability, sustainability, biocompatibility, biodegradability, functionality and renewability (Lizundia et al., 2022).
In spite of its promising properties, the conventional extraction and processing of chitin involves the use of chemicals with significant environmental impact, such as highly concentrated acidic and basic solutions (Maddaloni et al., 2020). Therefore, our aim is to explore more environmentally friendly alternatives, and give specific numbers on the environmental impacts of currently applied processes (Figure 1).
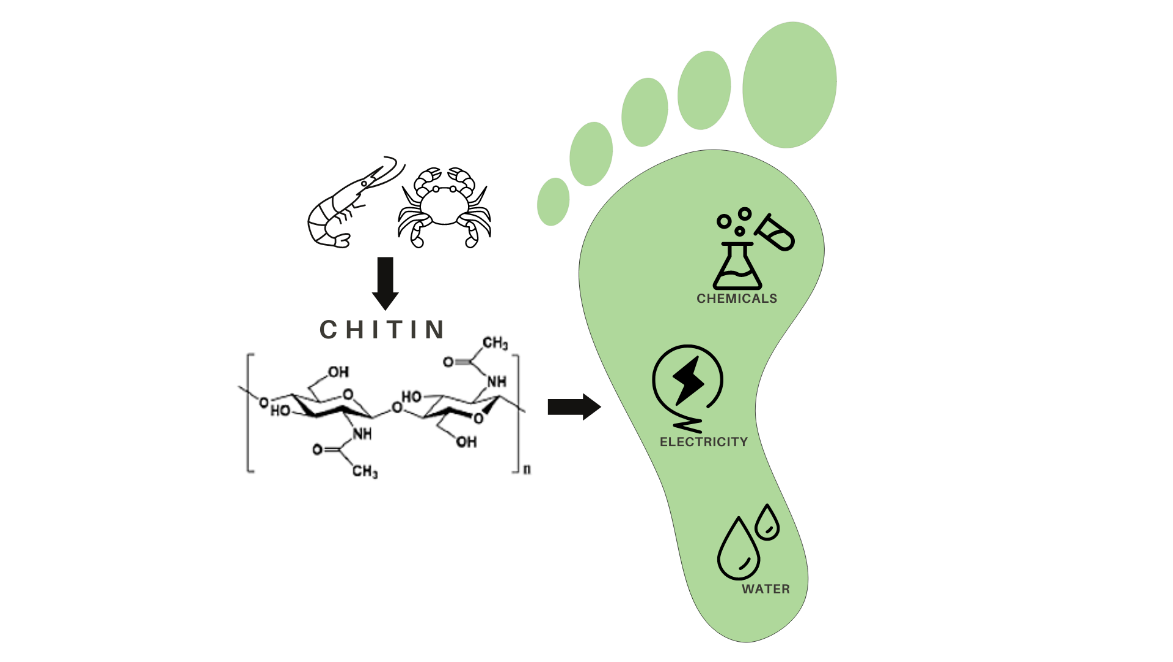
Conventional extraction of chitin and chitosan
Due to the abundance of waste crustacean exoskeletons, extraction from crustaceans is the most common procedure, and two routes can be generally followed: biological, or chemical methods (see Figure 2). Both of them require two fundamental steps: demineralization and deproteinization.
During the biological method, demineralization occurs due to lactic acid produced by lactic acid bacteria. Lactic acid reacts with the calcium carbonate (CaCO3) present in the exoskeleton of crustaceans, leading to the formation of calcium lactate, which precipitates and could be then removed. In addition, lactic acid also provides the low pH needed to activate the protease present in the biowaste, which is responsible for the deproteinisation step. Although the biological method can be regarded as a relatively environmentally friendly, it is a slow process (on average 6-7 days, and a maximum of 14 days) due to the use of lactic acid bacteria (for demineralization) and proteases (for deproteinization). For this reason, the chemical method is still the most widely used (Arbia et al., 2013).
The chemical method consists of several steps. The initially ground raw materials are treated with an acid solution (usually 1-to-2 M HCl at nearly 100 °C) for up to 48 hours. The aim is to remove the mineral components of the exoskeleton, which are calcium carbonate and calcium phosphate (Ca3(PO4)2). Subsequently, an alkaline treatment is applied to remove proteins from the demineralized shells. The proteins are removed upon the application of a NaOH treatment (usually 1 M, 3-6 hours at 65-100 °C), resulting in the production of chitin. Successive freshwater washing steps to achieve neutralization can follow all these steps. Unfortunately, these washing steps require large amounts of water, which further limits the sustainability of the whole process (Maddaloni et al., 2020). In addition, chitin can be transformed into chitin nanocrystals (ChNC) through an additional process of acid hydrolysis (Yang et al., 2020).
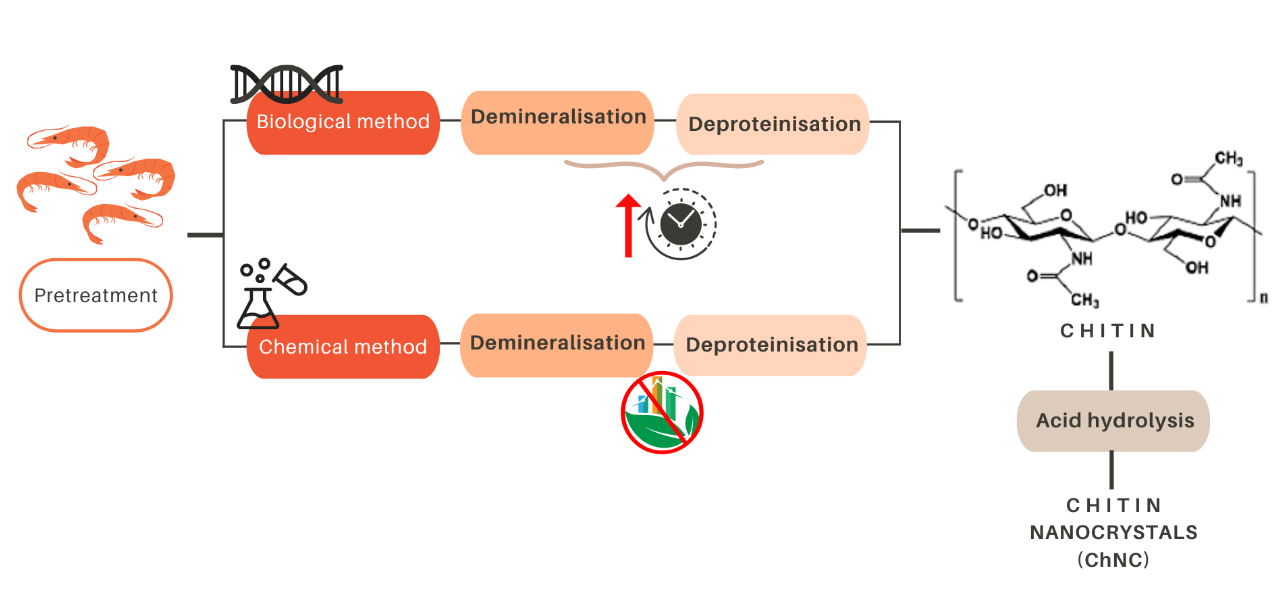
Possible applications
In recent years, chitin and its derivatives have attracted great interest in various applications due to their excellent biocompatibility, biodegradability, and mechanical/thermal properties. Moreover, not only can they be easily transformed into films, hydrogels, microspheres, or aerogels, but, as they present a highly organized micro- and nanofibrillated structure, it is possible to obtain nanochitin (nanocrystals and nanofibres) (Bai et al., 2022).
These biopolymers are nowadays used in the biomedical and pharmaceutical industry. For example, in drug delivery, wound dressing, bone regeneration and tissue engineering. It has also been used in the agricultural, food and cosmetic industries as a pesticide, food additive, food preservative and antioxidant. In addition, its excellent biocompatibility and film-forming ability make this material ideal to be applied as electrochemical biosensors. From an environmental point of view, it can also be used as an adsorbent for heavy metals, pesticides and dyes, and can be used for water purification, filtration membranes and wastewater treatment. On the other hand, in agricultural production, it can be used as fertilizer carrier nanoparticles to reduce harmful effects on human and plant health (Vidal et al., 2022).
Novel chitin isolation routes in line with Circular Economy end sustainability principles
The use of strong acid or alkaline solutions, large amounts of water and long periods for neutralization steps are the main limitations of extraction protocols. In recent years, the Circular Economy has highlighted the need to find environmentally friendly alternatives for chitin extraction. In particular, deep eutectic solvents (DES) and natural deep eutectic solvents (NADES) have generated scientific interest thanks to their ability to dissolve and extract substances from natural sources. Considering their physical properties, they can be defined as a new class of ionic liquids that have the particular advantage of ease of preparation and availability from low-cost components (Vidal et al., 2022).
In particular, as summarized in Figure 3, Zhao et al. described an interesting two-step method to obtain chitin using different DES and citric acid combined with microwave heating. In this extraction process, well washed, dried and ground shells are treated with 10 % citric acid (a weak edible acid that can be extracted from natural sources) for demineralization (Zhao et al., 2019). Citric acid replaces HCl in the classical chemical extraction. The deproteinization step is performed by suspending the pre-treated samples in various DES solutions and heating them by microwave irradiation. Finally, chitin can be isolated from DES by simple centrifugation and can be reused up to 5 times without purification (Zhao et al., 2019). The main advantage of this method over the classical method is that not only chitin, but also the chemicals used for extraction can be obtained from food waste, vegetable and natural sources. In addition, the recyclability of DES or NADES makes the method compatible with the principles of a circular economy.
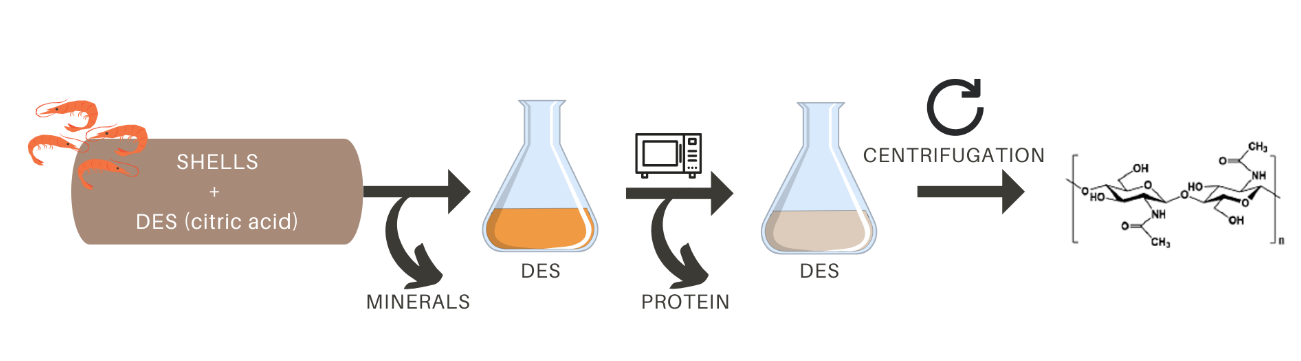
Life cycle assessment
In spite of the virtues of chitin extraction, which can use food waste as a resource, it remains unclear whether or not these processes are environmentally sustainable. In this sense, the life cycle assessment (LCA) methodology results a powerful methodology as it accounts for the environmental impacts originating from chitin isolation. Importantly, LCA offers a standardized way according to International Standards ISO 14040 and ISO 14044 for the quantification, disclosure, and comparison of the environmental impacts related to the life cycle of materials processes, technologies or even services. In this context, a recently published study by Berroci et al. compares conventional processes for the hydrolytic isolation of ChNCs using three different sources; shrimp shells, chitin powder and crab shells.
Eighteen impact indicators were analyzed by extending the laboratory quantities to processes treating 1 kg of biowaste. A global warming potential value of 906.8 kg-CO2-equiv.kg-1 is obtained for ChNC from shrimp shells, followed by 543.5 kg-CO2-equiv.kg-1 for ChNC extracted from crab shells and 105.2 kg-CO2-equiv.kg-1 for ChNC from chitin powder (Berroci et al., 2022). For comparison, the carbon footprint of commodity plastics such as polypropylene or polyethylene terephthalate remains nearly at 1-to-5 kg-CO2-equiv.kg-1. Other impact categories including acidification, water use or eutrophication were also considered. The chitin isolation from crustacean exoskeleton results the step mainly contributing to the environmental impacts.
Although the environmental impacts of novel extraction routes using DES or NADES have not yet been explored, it appears that, at least nowadays and in spite of its virtues, chitin nanocrystal isolation from marine food waste results a non-sustainable process; at least if we apply current valorization methods.
Conclusion
The growth of the world’s population has led to the search for new sustainable methods of disposal and reuse of food waste. In particular, the recognition that food waste can be a source of high value-added molecules (proteins, polysaccharides, lipids, etc.) has led to new research in the field of materials science, biotechnology and engineering.
Chitin and its derivatives are important examples of polysaccharides obtained from food waste, especially from crustaceans and fungi. Their recyclability, biodegradability and non-toxicity are the main advantages of their use in the preparation of advanced functional materials for various applications. However, these processes are not always environmentally sustainable and can bring serious environmental issues such as chemical use, wastewater generation and energy consumption. In particular, the use of strong bases and acids during the extraction process, the relatively high temperatures and long reaction times, and the large amounts of water used during washing steps remain important limitations that need to be addressed in the near future.
This work summarizes part of the research findings focusing on the use of alternative ecological pathways. These unconventional strategies are based on the use of green solvents and heat sources. The ability to purify and recycle these extraction chemicals provides the opportunity to develop full-cycle processes in which by-products from one step can be used as reagents in the next step, creating an inner cycle around the concept of “systems chemistry” and “circular economy”.
Acknowledgements
The authors are grateful for the financial support from the “2021 Euskampus Missions 1.0. Programme” granted by Euskampus Fundazioa and the University of the Basque Country (Convocatoria de ayudas a grupos de investigacion GIU21/010).
References
Arbia, W., Arbia, L., Adour, L., & Amrane, A. (2013). Chitin Extraction from Crustacean Shells Using Biological Methods – A Review, Food Technology and Biotechnology 51 (1) 12–25. https://hrcak.srce.hr/file/146860
Bai, L., Liu, L., Esquivel, M., Tardy, B. L., Huan, S., Niu, X., Liu, S., Yang, G., Fan, Y., & Rojas, O. J. (2022). Nanochitin: Chemistry, Structure, Assembly, and Applications. Chemical Reviews, 122(13), 11604-11674. https://doi.org/10.1021/acs.chemrev.2c00125
Berroci, M., Vallejo, C., & Lizundia, E. (2022). Environmental Impact Assessment of Chitin Nanofibril and Nanocrystal Isolation from Fungi, Shrimp Shells, and Crab Shells. ACS Sustainable Chemistry & Engineering, 10(43), 14280-14293. doi: 10.1021/acssuschemeng.2c04417
Lizundia, E., Luzi, F., & Puglia, D. (2022). Organic waste valorisation towards circular and sustainable biocomposites. Green Chemistry, 24(14), 5429-5459. doi: 10.1039/D2GC01668K
Maddaloni, M., Vassalini, I., & Alessandri, I. (2020). Green Routes for the Development of Chitin/Chitosan Sustainable Hydrogels. Sustainable Chemistry, 1(3), 325-344. https://doi.org/10.3390/suschem1030022
Roy, P., Mohanty, A. K., Dick, P., & Misra, M. (2023). A Review on the Challenges and Choices for Food Waste Valorization: Environmental and Economic Impacts. ACS Environmental Au. https://doi.org/10.1021/acsenvironau.2c00050
Vidal, J. L., Jin, T., Lam, E., Kerton, F., & Moores, A. (2022). Blue is the new green: Valorization of crustacean waste. Current Research in Green and Sustainable Chemistry, 5, 100330. https://doi.org/10.1016/j.crgsc.2022.100330
Yang, T., Qi, H., Liu, P., & Zhang, K. (2020). Selective Isolation Methods for Cellulose and Chitin Nanocrystals. ChemPlusChem, 85(5), 1081-1088. https://doi.org/10.1002/cplu.202000250
Zhao, D., Huang, W.-C., Guo, N., Zhang, S., Xue, C., & Mao, X. (2019). Two-Step Separation of Chitin from Shrimp Shells Using Citric Acid and Deep Eutectic Solvents with the Assistance of Microwave. Polymers, 11(3), 3. https://doi.org/10.3390/polym11030409