Nanohazards
Just a few years ago, nanoparticles broke into almost every laboratory in the world in one way or another. It marked a boom in nanoscience and nanotechnology. The demand for these new ultrafine particles, halfway between the bulk scale and the molecule scale, increased in such a way that sometimes there was even a shortage of them in order to implement new industrial processes and products. In some way, everything with the added prefix “nano” became trendy. Scientific publications which long before had been dedicated to these small particles and their applications underwent a remarkable increase in their impact factors. The media started echoing the wonders of nanoparticles. Research groups in many different areas felt the need to enter this new field of nanoscience. We might well say that a bubble of the “nano” was emerging.
However, while nanoscience and nanotechnology have undergone this exponential growth, the study of the toxicology of nanoparticles is still in its very early stages, and this is far from being a good practice in the long term. The concerns are justified due to our previous experience with health risks caused by the release into the environment of other ultrafine particles such as asbestos and air pollution. The long-term exposure to these particles via the airways has proved critical in accounting for some respiratory diseases and an increase in mortality.
The key point to be taken into account is that the toxicology in the nano-scale is not governed by the same laws as conventional toxicology. While in bulk materials concentration and time are the main parameters to risk assessment, when matter is reduced to smaller pieces new phenomena arise due to the effect of an increased surface-to-volume ratio. Unfortunately, there is a lack of knowledge about the potential hazards of the interaction between nanoparticles and living systems, and that’s why there is an urgency to understand the mechanisms that operate in nanotoxicology. At the moment, the use of engineered nanoparticles, that is, nanoparticles created in the laboratory for different applications, is practically unregulated. Nevertheless, there is a growing number of industrial processes and products in which they are being introduced and therefore an increase in human exposure and release into the environment 1.
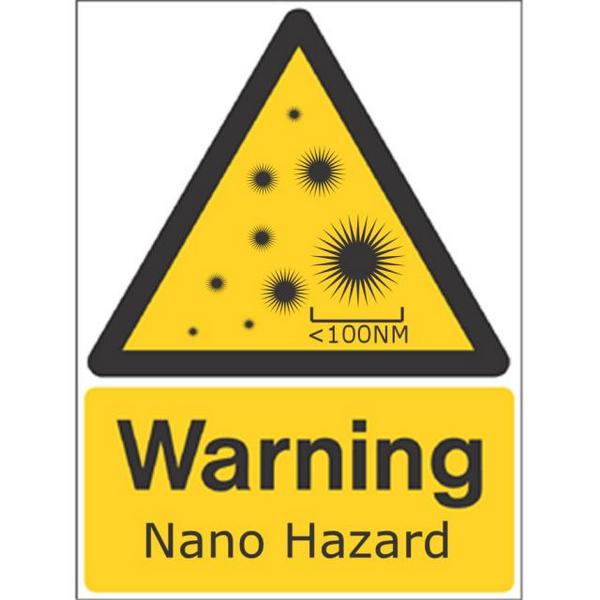
Of course there is no doubt about the numerous benefits derived from the use of nanoparticles in different fields of application. They have been successfully introduced in conventional materials leading to exceptional improvements in mechanical, thermal and electrical properties, fire resistance, antimicrobial effects and so forth. Furthermore, perhaps the most amazing application is in the biomedical field. Their ultra small size has made their use possible in areas in which traditional therapeutics had failed, such as drug delivery into targeted tissues and cells, cancer therapy or tissue engineering. But even in these applications, little is known about what happens to these nanoparticles within the body once they are no longer in use2.
The Nano Systems Biology Group from the Centre for Molecular Biosciences at the University of Ulster has recently published a review about nanotoxicology3 in which they have successfully tried to get together the main findings in this field so far, as well as the path to follow towards proper hazard identification of human exposure to engineered nanomaterials. It seems that airborne, unbound nanoparticles raise the most concern from a toxicological point of view. The main entry routes of nanoparticles into the body are via the airways, the skin or via digestion, the most likely being the respiratory system. Once they have gained access to the airways and lungs, the main question to be solved is whether they can cross the barrier and get into the bloodstream, moving from the lungs to the rest of the body. Although less likely, the skin and the digestive system could also be affected due to the increasing use of nanoparticles in cosmetics (e.g. titania nanoparticles are used as additives for sunscreen products) or as food additives.
The small size of nanoparticles makes it really difficult to assess their toxicology. The very well known phrase by Paracelsus “the dose makes the poison”, takes on a special meaning when dealing with the nanoscale. While traditionally the dose is related to the mass of the substance, it seems that for nanoparticles the dose is directly related to the total surface in contact, and this is a very difficult parameter to evaluate.

As the particles become smaller, the surface-to-volume ratio increases, leading to a change in the surface chemistry and an increase in their chemical reactivity. This factor is crucial for the interaction of nanoparticles with living systems. In a biological environment, nanoparticles tend to attract smaller structures such as atoms, atom clusters, single molecules and macromolecules, that get attached to the surface of the nanoparticles leading to a surface layer called “corona” (figure 2). As a result, the environment surrounding the nanoparticles plays a major role in understanding the mechanisms by which nanoparticles can cause toxicity when moving inside the body, as they can transport contaminants.
In vitro nanotoxicology studies have confirmed that engineered nanoparticles could behave in the same way as viruses do when they are in contact with living cells. They can cross cell’s membranes via endocytosis and then they can affect different parts within the cell (figure 3). The toxicological mechanisms due to the interaction between biological systems and nanoparticles can be either physical or chemical. The most important of them is the production of reactive oxygen species (ROS), which is involved in inflammatory processes and can also cause cell damage and even cell death. Apart from this one, other harmful processes such as release of toxic ions, disruption of membranes activity or protein misfolding can define the “biological response” to nanoparticles.

There are grounds for thinking that nanoparticles’ surface properties are decisive in order to evaluate the interaction with cell membranes, and a wider knowledge of these properties will only be possible if a thorough characterization of the nanoparticles is carried out. A proper characterization will allow the design of suitable surfaces which reduce the potential damage to living cells. This knowledge is essential in the field of biomedicine, when nanoparticles are deliberately introduced inside the body.
These in-vitro results are useful in order to define the potential risks of nanoparticles for different biological systems, but there is an urgent need for in-vivo and epidemiological studies to predict the possible hazards affecting humans as a result of the increasing use of these new particles. The entry routes (lung, gut and skin) and the putative targets (lung, liver, heart and brain) have been identified, but there is still a long way to go before proper methodologies are developed to assess the long term toxicological effects and to elaborate a regulatory framework for the use of nanoparticles. Nonetheless, people in contact with ultrafine particles, specifically workers and researchers, have to be especially cautious when handling them, as they are the people who are most exposed to the possible dangers at the moment4.
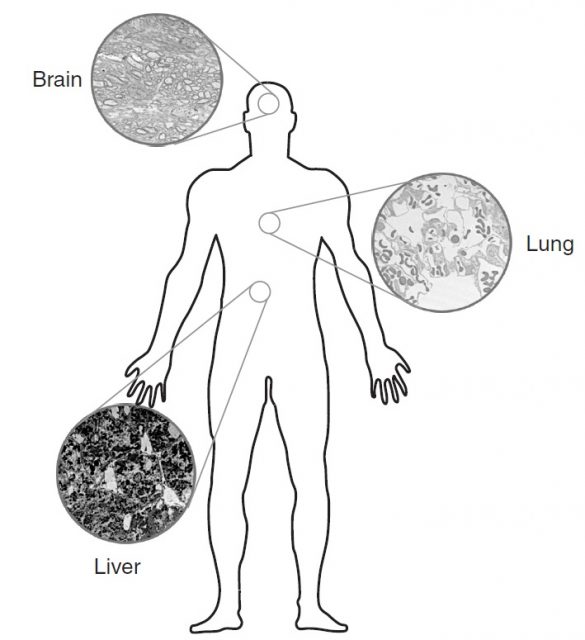
References
- Zhang R., Bai Y., Chen L., Yan B., The potencial health risk of titania nanoparticles, Journal of Hazardous Materials, 211-212 (2012) 404-413. doi: 10.1016/j.jhazmat.2011.11.022 ↩
- Radad K., Al-Sharaim M., Moldzio R., Rausch W., Recent advances in benefits and hazards of engineered nanoparticles, Environmental Toxicology and Pharmacology, 34 (2012) 661-672. http://dx.doi.org/10.1016/j.etap.2012.07.011 ↩
- Elsaesser A., Vyvyan Howard C., Toxicology of nanoparticles, Advanced Drug Delivery Reviews 64 (2012) 129-137. doi: 10.1016/j.addr.2011.09.001. ↩
- Liou S.-H. et al, Epidemiological study of health hazards among workers handling engineered nanomaterials, Journal of Nanoparticle Research, (2012) 14: 878. doi 10.1007/s11051-012-0878-5. ↩
1 comment
[…] The unique properties of superparamagnetic nanoparticles have found numerous applications in the biomedical field, such as tissue repair by local heating, detoxification of biological fluids, magnetically controlled delivery of drugs or genes, or as contrast agents in magnetic resonance […]