Getting the best grip: how ridged surfaces affect finger pad tribology

Humans can build and use the most sophisticated tools. One of the reasons is the configuration of our hands, which enable us to hold items in a very convenient way. But further than that, we can actually grab items because of the friction coefficient that exists between the skin of our hands and the surface of the element we are picking up. For instance, grabbing a piece of ice might be a frustrating task, as its friction coefficient is very low and we cannot exert the necessary tangential forces in the contact surface to keep it fixed to our hand.
Another daily life example of hand-to-tool sliding (and consequent frustration) is when you try to open the screw cap of a can. It is not that the friction coefficient is not high enough (you can grab it pretty easily when it is already opened), but the pre-load of the cap against the glass is higher than the tangential friction torque that you can exert. The friction force limit (known as Coulomb’s limit) is proportional to the exerted normal force and the friction coefficient, so in order to open the can you can increase either of them, e.g. using a wet cloth to increase the coefficient of friction.
Some items, in order to improve the grip between the hand and the tool, introduce a modification in the surface, usually in the shape of ridges. Soda bottles with vertical ridges in the cap, metal tools with cross-ridged surface for dentists, sports equipment… there are several applications were ridged surfaces are used. However, there is a high variability in the characteristics of these ridges, with heights between 0.1 and 5 mm, widths between 0.2 and 9.5 mm, and spacing between 0.2 and 5.5mm 1. The characteristics of these textures are usually driven by design interests, focused on “how does it feel” more than “is its performance optimal?”
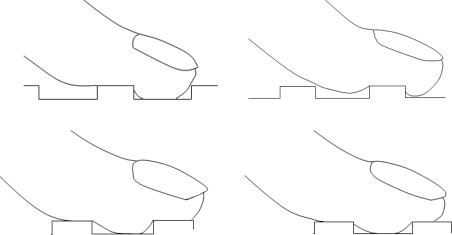
The study of the tribology and mechanics of tool-grabbing is important because, according to HSE (the national health regulator in UK), there were more than 40 000 accidents reported between 2007 and 2008 due to “handling, lifting or carrying”. Understanding how the grip of the tools can be optimized might prevent some of these accidents. Moreover, improving the grip can increase their performance, e.g. increasing precision of surgical tools or making home tools friendlier for elderly or disabled people. However, there were only very limited experimental analyses of how the characteristics of the ridges affect the tribology of the grip. In a recently accepted paper, Tomlinson et al. 2 have performed an extensive analysis of the tribological properties of a wide variety of rectangular ridged patterns.
In these experiments, a volunteer was asked to slide the finger along the ridged surface with constant normal pressure, and the normal and tangential forces were measured. In practice, it is very difficult to maintain this pressure, because when the finger jumps into the gap the normal pressure is lost, and when it slides onto a ridge the pressure increases, as it can be seen in Figure 2.
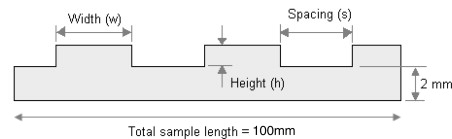
In this particular case, there are two friction mechanisms that are added to the overall friction value: dry friction, which accounts for the energy required to slide tangentially two parallel surfaces; and ploughing friction, which accounts for the energy required to deform an elasto-plastic surface in order to continue with the tangential sliding. This second type of friction occurs when the finger jumps onto a protrusion, and part of this is returned by the elastic behaviour of the finger when it goes into the next ridge.
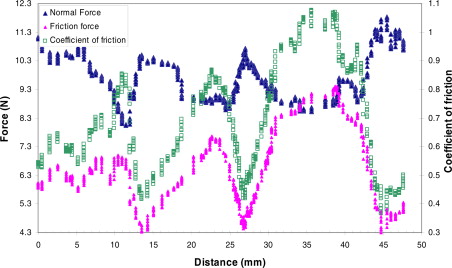
As a side effect of the variation in the normal force, the friction coefficient has a huge variation. The technical solution for the analysis of the results is to compare the maximum and minimum values of the friction coefficient, as seen in Figure 4.
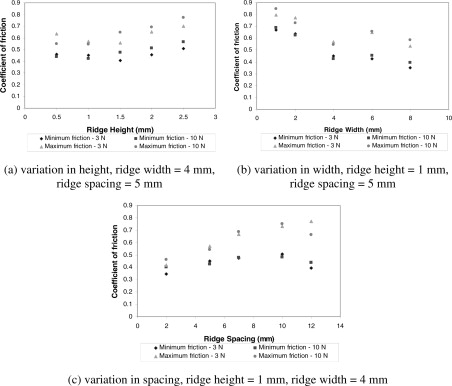
In all the studied cases, they have found that the friction lost by the reduction of the contact area is compensated (and even surpassed) by the ploughing friction, so the overall result is that ridges increase the friction coefficient in most of the cases. Figure 4 also depicts the individual influence of each of the ridge variables in the coefficient of friction:
- Considering the depth of the ridge, the ploughing friction increases with the volume of finger that we have to deform, so for a higher depth we get a higher friction value.
- The friction coefficient decreases with the width of the ridge, but only until 4mm. This makes sense, as we said that the ploughing friction has more influence than the dry friction, and increasing the top surface of the protrusions will increase the dry friction and decrease the ploughing.
- For the separation of the ridges, it is pretty clear that for more separation there will be more space for ploughing, until some point at 10mm when it cannot be increased anymore. Then the dry friction starts to have more influence and, thus, the overall friction is slightly reduced.
In my opinion, the design of the experiment is limited, but good enough for a preliminary analysis. The dimensions of the analysed ridges are too big compared to the functional ridges in the commercial tools and instruments, which has both good and bad characteristics. It is good as the mechanism of ridges can be clearly visualized and stated; and bad because with ridges one order of magnitude smaller, the nonlinear elasto-plastic properties of the finger pad might have a different influence on the surfaces in contact.
References
- S.E. Tomlinson, Understanding the friction between human finger and contacting surfaces. PhD Thesis, The University of Sheffield, Department of Mechanical Engineering, 2009. ↩
- S.E. Tomlinson, R. Lewis, M.J. Carré, S.E. Franklin, Human Finger Friction in Contacts with Ridged Surfaces, Wear, Available online 18 January 2013, ISSN 0043-1648, 10.1016/j.wear.2012.12.039. ↩
2 comments
Sorry, but can you please reupload the pictures? they are all down.
Reuploaded. Thank you for the heads up.