The perfect armour
If you have ever faced that thankless task of cleaning fish, for sure you will have realized, at least envisaged, the complex architecture of the fish’s natural armour. Fish scales play the role of a skeleton which provides both protection and flexibility at the same time. It goes without saying that this fact has not gone unnoticed by materials engineers, who have rapidly started to think about new bioinspired materials.
As many other bone-like biomaterials, scales comprise an organic component, consisting of collagen fibers, and a mineral component, which provides mechanical resistance. This system has been studied extensively and some interesting conclusions have been reached. It seems that each individual scale has a highly ordered structure in which the collagen fibers are arranged in layers of about 2 microns thick. These fibers are carefully coated by crystals of calcium-deficient hydroxyapatite – the mineral phase 1. Apart from these characteristics common to almost all fish, it is noteworthy that different species of fish possess scales which present variety in structure, composition and shape depending on their specific requirements.
New approaches have described fish scales as a nanocomposite laminate structure, in which nano-crystals of hydroxyapatite act as reinforcement of the collagen matrix 2. This sophisticated design, together with the scales assembly along the fish’s skin, results in a perfect combination of mechanical resistance and flexibility. However, up to now the description of the mechanical properties of this complex biomaterial has just been focused on scale response to blunt trauma or indentation.
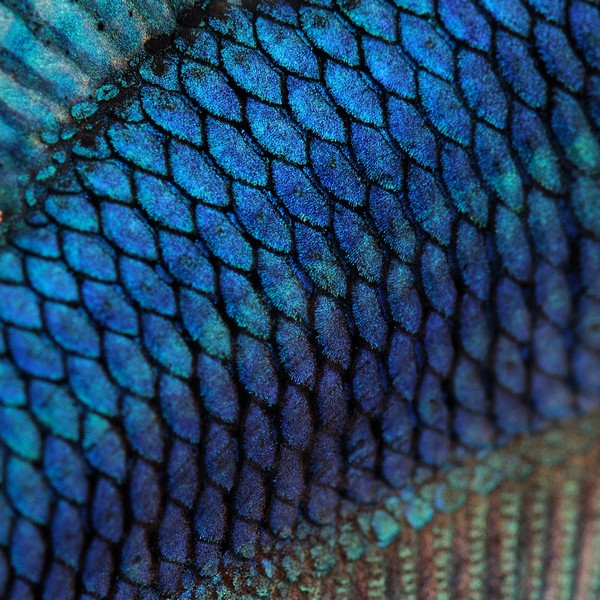
According to a recent study carried out by researchers at the Department of Mechanical Engineering at the Massachusetts Institute of Technology (MIT)3, in order to gain a more comprehensive approach to the mechanical behaviour of fish scales we must consider the scales together with the soft non-mineralized tissue in which they are embedded. This new system (scales-soft tissue) explains, in a more accurate way, the excellent penetration resistance and flexibility of this biomaterial. Actually, the combination of soft tissues and mineralized components is very usual in biological systems. The study concludes that the geometric arrangement of both the scales and the underlying tissue governs the deformation mechanisms of the resulting structure.
In order to fully understand the mechanical behaviour of this dermal armour, Browning et al. have developed a three-dimensional prototype consisting of two different engineering polymers with similar mechanical properties to those of the biological materials. The scales were substituted by acrylonitrile butadiene styrene (ABS), while the soft tissue was substituted by a silicone rubber. This prototype was fabricated in a variety of geometric configurations (figure 2). Furthermore, a scale-tissue micromechanical model was also simulated using finite element analysis.
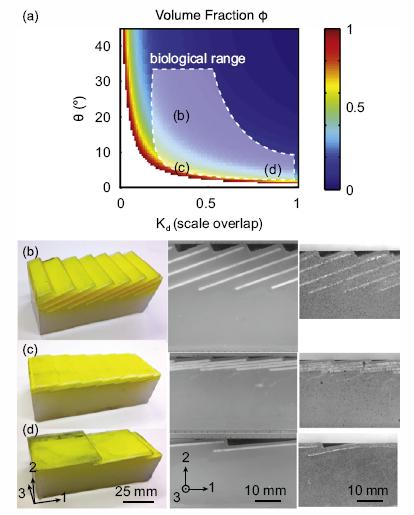
It seems that the mechanical response to blunt loading and indentation can be tailored by four different structural parameters:
-
The degree of imbrication or scale overlapping (Kd), meaning the ratio between the exposed part of the scale and the part embedded beneath the next scale.
-
The initial scale angle (θ) relative to the soft tissue support.
-
The volume fraction (ϕ), which represents the scale-to-tissue ratio in the upper layer, not including the underlying soft tissue.
-
The aspect ratio (Ra) of the scale, the ratio between length and thickness of the scale.
Varying these parameters allows the design of a wide range of materials with different degrees of protection and flexibility. The importance of considering the underlying tissue lies in the deformation mechanisms involved under loading conditions. Tissue constraint and tissue shearing are essential to provide flexibility to the whole structure. Together with tissue constraint and tissue shearing, the other deformation mechanisms are scale bending and scale rotation, the latter taking place only under tissue shearing.
The study has found that under a blunt non-penetrating loading, scale overlapping (Kd) governs the scale bending, distributing stresses in a more effective way than in a continuous armour layer. The volume fraction () governs tissue constraint, while scale angle () is responsible for tissue shearing and subsequent scale rotation. Moreover, adjusting the scale aspect ratio (Ra) allows the changing of the deformation mechanism from scale bending to scale rotation by tissue shearing.
On the other hand, as the contact area of the loading surface decreases, simulating a tooth indentation, scale bending is the main deformation mechanism and it is dominated by scale overlapping. The higher the scale overlap, the better the loading distribution over a large area, avoiding the risk of scale fracture and tissue injury.
To sum up, the main contribution of this new approach to the description of fish scales mechanical behavior is that the interplay between scales and the underlying tissue has been taken into account. This interaction is key to understanding not only the way in which nature cleverly manages to bring about a perfect combination of protection and flexibility, but also our ability to copy this architecture in new biomimetic materials.
In fact, this kind of design has been already used in protective systems, such as in the defeating of high-velocity projectiles4, elastic composite textiles5, and even in electronics 6. The future is likely to hold the development of multiple applications in different fields, the most attractive of which may well be in the field of medicine. We will just have to wait and see.
References
- Ikoma, T., Kobayashi, H., Tanaka, J., Walsh, D., Mann, S., Microstructure, mechanical and biomimetic properties of fish scales from Pagrus major. Journal of Structural Biology 142 (2003) 327-333. ↩
- Torres, F.G., Troncoso, O.P., Nakamatsu, J., Grande, C.J., Gomez, C.M., Characterization of the nanocomposite laminate structure ocurring in fish scales from Arapaima Gigas. Materials Science and Engineering C 28 (2008) 1276-1283. ↩
- Browning, A., et al., Mechanics of composite elasmoid fish scale assemblies and their bioinspired analogues. Journal of the Mechanical Behaviour of Biomedical Materials (2013), http://dx.doi.org/10.1016/j.jmbbm.2012.11.003 ↩
- Neal, M.L., Bain, A.D., Method and apparatus for defeating ballistic projectiles. US Patent 6745661, 2004. ↩
- Hatjasalo, L., Rinko, K., Elastic composite structure. US Patent 7153789, 2006. ↩
- Kim, S., Su, Y., Mihi, A., Lee, S., Liu, Z., Bhandakkar, T.K., Wu, J., Geddes III, J.B., Johnson, H.T., Zhang, Y., Park, J.K., Braun, P.V., Huang, Y., Rogers, J.A. Imbricate scales as a design construct for microsystem technologies. Small, 8 (2012) 901-906. ↩