Some non-biological materials move as proteins do: the role of water
Proteins were traditionally described as immobilized objects and classified according to the chemical composition (amino acid sequence) or the three-dimensional structure. However, in the seventies, with the idea that “If nothing can move, nothing can function”, Perutz 1 first described the movements hemoglobin must undergo to fulfill its function and after him Frahuendelder 2 revealed a hierarchical organization of protein motions and the existence of multiple conformational substates.
Today, it is accepted in both physics and chemistry communities that protein dynamics spans a large hierarchy of timescales, from picoseconds to milliseconds or even longer. These motions of the protein (i.e. dynamics) include motions of the protein itself (such as vibrations, rotations, and transitions between different substates) as well as motions in the hydration shell and the bulk solvent and all these motions are necessary for the functionality of the proteins. However, in the absence of the solvent (dehydrated state), these motions are not produced. Therefore, water is seen as the ‘matrix of life’ because its presence is essential for every bio-molecular process (Figure 1). Furthermore, it is also accepted that the most important protein motions follow temperature dependences similar to the water motions 34. In the current literature this is called “slaving behavior” in the sense that the protein motions are coupled to motions in the surrounding solvent. However, this “slaving behavior” was exclusively observed in protein solutions and not in other non-biological systems.
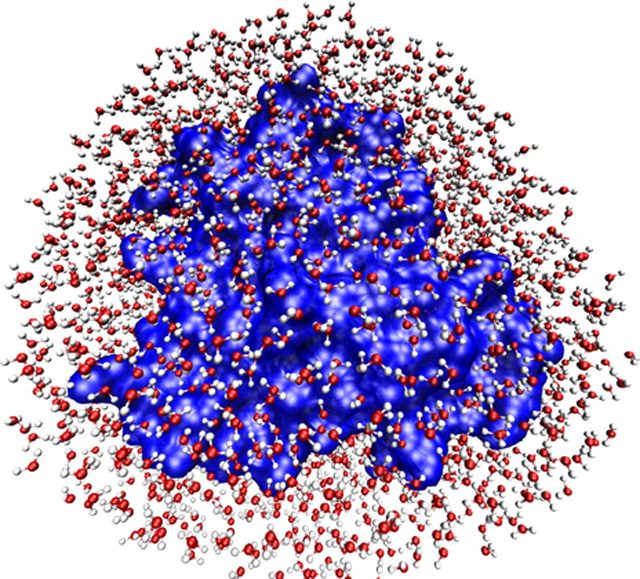
Now, a team of researchers from CFM (CSIC-UPV/EHU), DIPC and Chalmers University of Technology, have revealed that this slaving behavior is not only produced in protein solutions. Instead, this behavior can be also observed in non-biological solutions of amino acids and small peptides 5. These small molecules have no biological function, but nevertheless show the typical slaving behavior as proteins exhibit.
To measure the time for reorientation of water and solute molecules we have used dielectric spectroscopy (a technique which measures the dipole reorientation of the molecules in a wide temperature and frequency range). Normally, to access the relaxation times, these measurements are performed at low temperatures (from 100 to 300 K) and therefore it is necessary to find an appropriate system to elude crystallization on cooling. We have avoided the problem of crystallization by reducing the size of the molecules and investigated aqueous solutions of n-lysine (n = 1, 3, 4 and 10) of different molecular weights and -polylysine (n =32). As shown in Figure 2, by measuring the relaxation times of both the solute and the solvent, we show that both follows the very same temperature dependence, although the relaxation times of the solute molecules are slower than those of the solvent. Even more, the collective character of the solute relaxation comes from the cooperativity of the water relaxation.
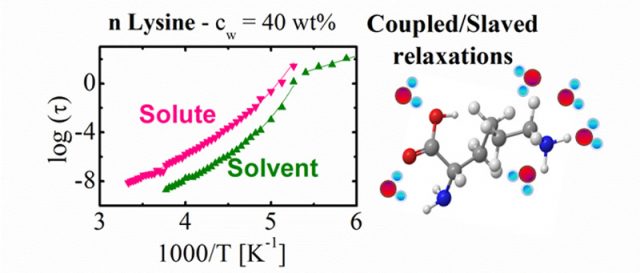
These observations suggest that the slaving phenomenon is more common than was previously thought 6 and the crucial role of water for protein dynamics can be extended to other types of macromolecular systems, where water is also able to determine their conformational fluctuations. This investigation also opens a new route to understand the slaving behavior observed for proteins.
Authors: Silvina Cerveny is a researcher at (CSIC, UPV/EHU)-Materials Physics Center (MPC) and DIPC, and Jan Swenson is a professor at Chalmers University of Technology
References
- Stereochemistry of cooperative effects in haemoglobin. Perutz, M. Nature (1970) 228, 726. doi:10.1038/228726a0 ↩
- The energy landscapes and motions of proteins. Frauenfelder, H., et al. Science (1991) 254, 1598. DOI: 10.1126/science.1749933 ↩
- Bulk-Solvent and Hydration-Shell Fluctuations, Similar to Alpha- and Beta-Fluctuations in Glasses, Control Protein Motions and Functions. Fenimore, P.et al, PNAS (2004), 101, 14408. DOI:10.1073/pnas.212637899 ↩
- A Unified Model of Protein Dynamics. Proceedings of the National Academy of Sciences of the United States of America. Frauenfelder, H et al (2009), 106: 5129. DOI: 10.1073/pnas.0900336106 ↩
- Evidence of coupling between the motions of water and peptides. Cerveny et al. J. Phys. Chem. Lett., (2016) 7:4093. DOI:10.1021/acs.jpclett.6b01864 ↩
- Spotlights. J. Phys. Chem. Lett. (2016) 7:4217. DOI:10.1021/acs.jpclett.6b02338 ↩