Quantum atomic fluctuations stabilize the crystal responsible for superconductivity at 250 K
Achieving room temperature superconductivity is among the most pursued but elusive goals of scientists. In December 2014 researchers claimed to have observed superconductivity as high as 200 K in hydrogen sulfide at high pressure, breaking all the records thus far. This meant that cuprates could be knocked from their position as the highest temperature superconductors. Moreover, the observation was revolutionary because it confirmed experimentally for the first time the theoretical intuition by Ashcroft that high-temperature superconductivity could be attained in metallic hydrogen or hydrogen-rich materials. Ion Errea explained this discovery in detail in Mapping Ignorance.
The recent synthesis of lantanum superhydride (LaH10) with a superconducting critical temperature (Tc) of 250K places these materials at the verge of reaching the long-dreamed room-temperature superconductivity. But, how does this work? What makes the material a superconductor? To know this is essential in our quest for superconductivity at room temperature. Ion Errea himself is the first author of a study published in Nature 1 that shows that quantum atomic fluctuations stabilize a highly symmetrical crystal structure that would be responsible for the superconductivity observed at 250 kelvin.
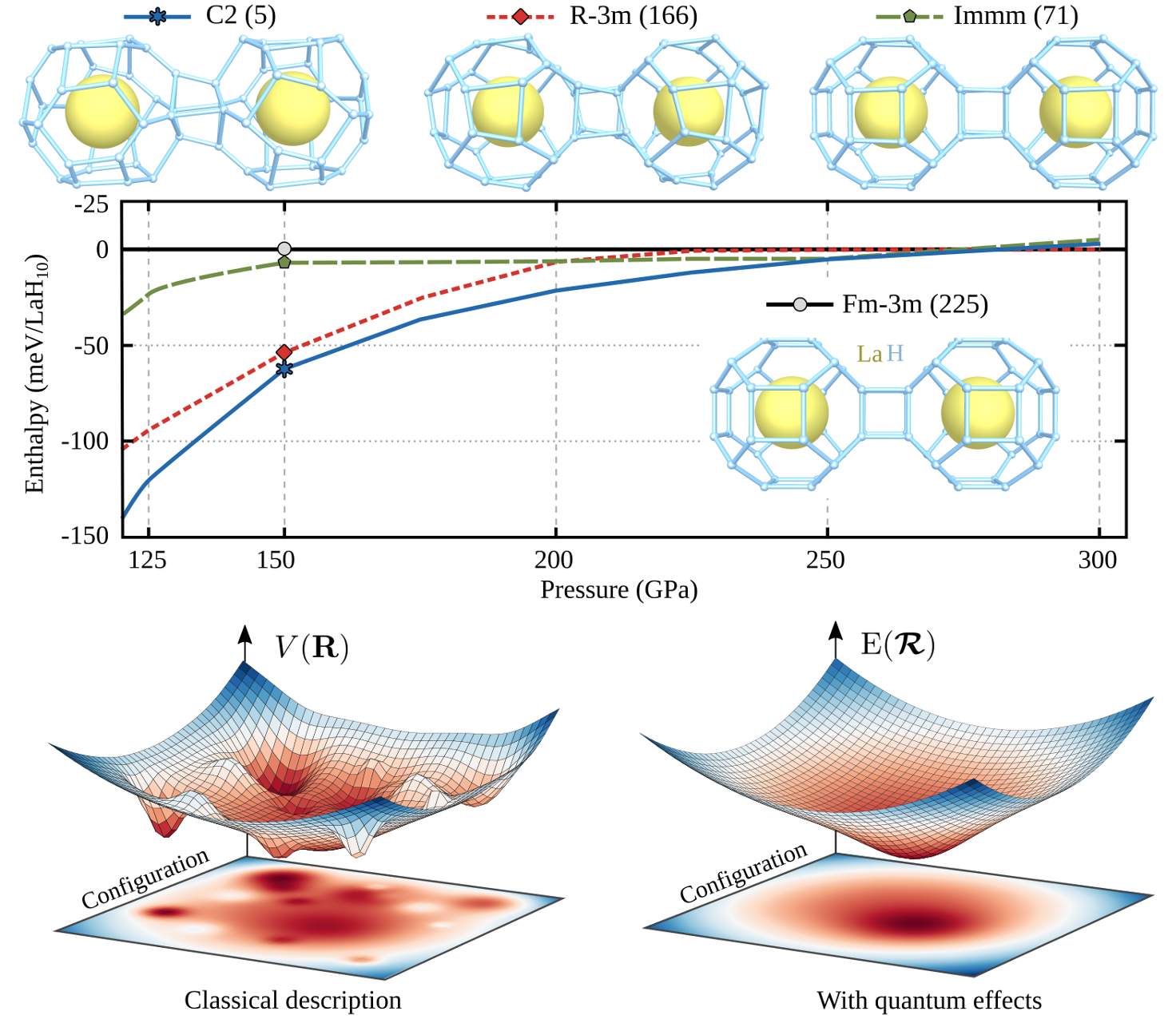
The band theory of metals has been subjected to experimental tests many times and is now the accepted model of the behaviour of conductors and insulators. But it has to be modified when it comes to superconductivity. Electrical resistance is due to collisions of the electrons (whether treated as particles or waves) with impurities, imperfections, and especially the lattice vibrations of the metal crystal. The lattice vibrations of the solid will decrease as the temperature falls, because the entropy, which represents disorder, also decreases. Therefore, the resistance should also decrease. This is what is observed, and it is well explained by quantum band theory.
But at very low temperatures, something strange happens: the resistance of some materials suddenly drops to zero at a certain temperature. These materials become superconductors, meaning they can conduct currents without resistance or loss of electric energy. The temperature at which superconductivity occurs is Tc.
The development of superconducting devices was greatly stimulated after the acceptance of the basic theory of superconductivity proposed in 1957 by John Bardeen, Leon Cooper, and Robert Schrieffer. The authors of the BCS theory, as it is known, received the Nobel Prize for their work in 1972. Their theory is highly technical but the basic idea is that the electron waves in the superconducting state no longer act independently, as in Bloch’s model. Instead, they are paired together at Tc so that their wave functions act as one unit as they interact with the crystal lattice (this is called electron-phonon coupling). Moreover, all the electron pairs move together in one collective motion, so that if any single electron is scattered by the lattice it is pulled back into the flow by its partner, and if any pair of electrons is somehow scattered off track, it is pulled back into the collective flow by all the other pairs. Since there is no scattering or inelastic collisions, there is no resistance, and the material becomes a superconductor.
The potential of metallic hydrogen as a high- Tc superconductor was identified few years after the development of the BCS theory. The main argument was that Tc can be maximized for light compounds due to their high vibrational frequencies.
The use of modern ab initio crystal structure prediction methods based on density-functional theory (DFT) has helped significantly predict hundreds of hydrogen-rich compounds to be thermodynamically stable at high pressures and, by calculating the electron-phonon interaction parameters, their Tc’s could be estimated. The success of this symbiosis between DFT crystal structure predictions and Tc calculations is exemplified, precisely, by the discovery of superconductivity in H3S at 200 K.
This theoretical prediction power make the prospects for discovering warm hydrogen-based superconductors in the next years high, in clear contrast with other high-Tc superconducting families such as cuprates or pnictides, where the lack of a clear understanding of the superconducting mechanism makes the search closer to a trial and error effort.
DFT predictions in the La-H system proposed LaH10 to be thermodynamically stable against decomposition above 150GPa. Early this year, evidences of a superconducting transition at 260K and 188GPa were reported in a lanthanum superhydride. These findings were confirmed and put in solid grounds few months later by an independent group that measured a Tc of 250K from 137 to 218GPa in a structure with face-centered cubic arrangement of the La atoms and suggested a LaH10 stoichiometry.
What Errea et al. show now is that quantum atomic fluctuations stabilize in all this pressure range a high-symmetry Fm-3m crystal structure – with a colossal electron-phonon coupling – consistent with experiments. Even if ab initio classical calculations neglecting quantum atomic vibrations predict this structure to distort below 230GPa yielding a complex energy landscape with many local minima, the inclusion of quantum effects simplifies the energy landscape evidencing the Fm-3m as the true ground state. The agreement between the calculated and experimental Tc values further supports this phase as responsible for the 250K superconductivity.
The relevance of quantum fluctuations in the energy landscape found here questions many of the crystal structure predictions made for hydrides within a classical approach that at the moment guide the experimental quest for room-temperature superconductivity. Furthermore, quantum effects reveal crucial to sustain solids with extraordinary electron-phonon coupling that may otherwise be unstable.
Author: César Tomé López is a science writer and the editor of Mapping Ignorance
Disclaimer: Parts of this article are copied verbatim or almost verbatim from the referenced research paper.
References
- Ion Errea, Francesco Belli, Lorenzo Monacelli, Antonio Sanna, Takashi Koretsune, Terumasa Tadano, Raffaello Bianco, Matteo Calandra, Ryotaro Arita, Francesco Mauri & José A. Flores-Livas (2020) Quantum crystal structure in the 250-kelvin superconducting lanthanum hydride Nature doi: 10.1038/s41586-020-1955-z ↩