Neutron sciences as an essential tool to develop ‘materials for a better life’
Until 1932, atoms were considered to consist in positive charges, called protons, located within the atomic nucleus, where the majority of the mass of the atom is concentrated, and negative charges, identified as electrons, surrounding the nucleus to make it electrically neutral. However, in 1932 James Chadwick discovered the neutron, an uncharged particle located at the nucleus with a similar mass of the proton. Thanks to this essential discovery, James Chadwick won the Physics Nobel Prize in 1935. However, it was not until a few decades later, that scientists began to make use of neutrons as probes to characterize matter, allowing Bertram N. Brockhouse and Clifford G. Shull to be awarded with the 1994 Physics Nobel Prize “for pioneering contributions to the development of neutron scattering techniques for studies of condensed matter” [1].
Since then, neutrons have been extensively used for materials characterization, complementary to X-rays. Both types of particles are very different in nature. X-rays are electromagnetic radiation composed by massless particles, called photons, with high energy and wavelengths ranging from 0.1 to 100 Å. Neutrons are particles with mass and no electric charge, showing wavelengths ranging from 0.0003 Å, for fast neutrons, to 495 Å, for ultracold neutrons. However, neutrons have smaller energies than X-rays, making them less destructive.
The way neutrons and X-rays interact with matter is also very different. X-rays interact with the electronic cloud of the atoms, and because of that, they interact better with heavy atoms, due to the bigger electronic clouds they possess with respect to lighter ones. This also means that they have many problems to probe lighter elements, which are characteristic of biological materials, where neutrons are essential to depict their structure and dynamics. In fact, neutrons interact with the nuclei of the atoms, and this interaction does not just depends on the atomic number (number of protons on the nucleus). This results into three key facts:
-
Neutrons can probe light elements, such as hydrogen, better than X-rays.
-
Neutrons can differentiate better atoms with close atomic numbers.
-
Neutrons can differentiate between isotopes, as they have different nucleus.
Further, neutrons have a magnetic moment, therefore allowing the study of magnetic structures in different ways (Figure 1).
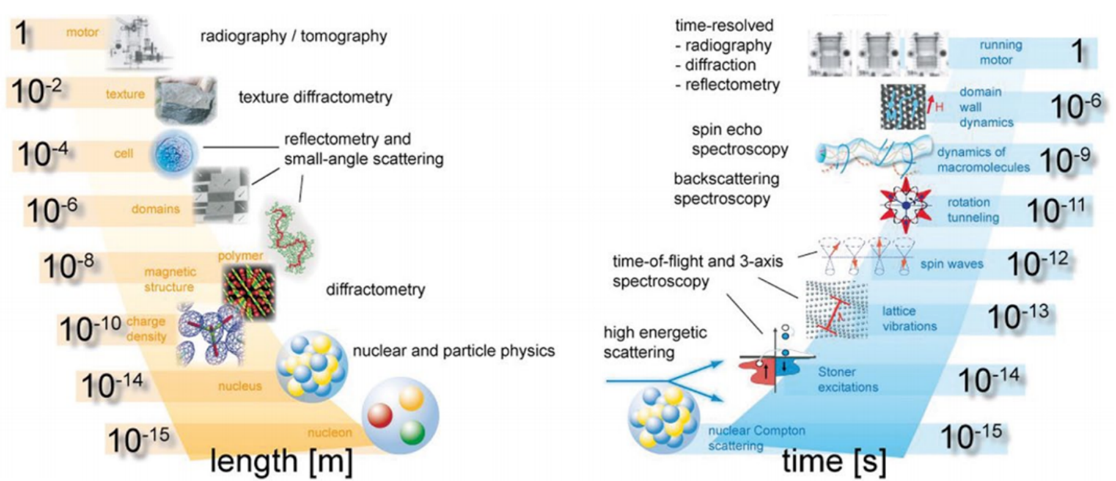
Nowadays neutrons are commonly used to investigate a wide variety of materials. In a typical neutron experiment, a neutron beam passes through the object under investigation, analyzing how the characteristics of the incoming beam change after its interaction with the sample. The variations in the beam passing through the sample allow scientists to obtain precise information about the internal structure and/or composition of the sample, according to the specific neutron scattering experiment [3].
The most widely used neutron scattering techniques are Neutron Imaging (NI), to evaluate the internal structure of materials; Neutron Diffraction (ND), for crystal structure determination; Small-Angle Neutron Scattering (SANS) and Neutron Reflectometry (NR), for large-scale structures (from the nanometer scale to microns) characterization, and Inelastic (INS)/Quasielastic (QENS) Neutron Scattering, to study atomic and molecular motions. Different methods provide different ways of achieving scattering patterns, and can be applied to different types of materials (Figure 1).
Neutron imaging is used to investigate the internal structure of objects with micrometric resolution. It is quite similar to X-ray (Radiography), which is typically used in medical applications. Since neutrons have large penetration depths and different materials attenuate neutrons differently, NI is widely used, for example, in the aerospace industry, by testing turbine blades of airplane engines or components for space programs, among others. NI is also an attractive method to analyze archaeological artefacts or pieces of art (Figure 2).
Neutron Diffraction is commonly applied to study crystalline solids, gasses, liquids or amorphous materials. The diffraction pattern resulting from neutron diffraction experiments can be recorded as a series of peaks of the scattered neutron intensity, which provides information about the position of the atoms and the distances among them.
Neutron Reflectometry and Small Angle Neutron Scattering are used for structural investigations of the planar and developed interfaces of materials. It is possible to probe structures at length scales from around 1 nanometer to more than 100 nanometers. NR and SANS have a wide range of applications from studies of polymers and biological molecules to nanoparticles, passing through microemulsions and liposomes, as well as layered nanostructures.
Inelastic and Quasielastic Neutron Scattering experiments analyze the change in kinetic energy of neutrons after passing through the sample. Motions of atoms or molecules are responsible for many of the characteristic properties of a material, including atomic momentum distributions, vibrations, and relaxation phenomena, among others. Thus, understanding the atomic and magnetic dynamics is essential in areas such as polymer sciences or information and communication technologies.
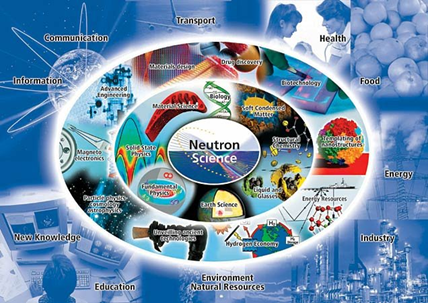
Examples of neutron science experiments contributions to science, technology and society cover a wide variety of disciplines across different scientific, engineering and biomedical areas. In the area of biological systems, neutron experiments play an essential role in determining their structure and behavior. The need to understand the structure and dynamics of biological macromolecules can be addressed by a combination of different neutron scattering techniques, such as INS/QENS, imaging or SANS. Pharmaceutical materials or supra-molecular chemistry, where hydrogen bonding plays an important role binding together complex molecules and larger structures, can be characterized by means of neutron diffraction and INS [5] /QENS [6], the latter being used to study molecular dynamics in chemistry [7].
Soft matter, where polymers, surfactants or liquid crystals are encompassed, are widely studied via neutron scattering experiments due to the suitability of the length and time scales that can be reached by neutrons, together with the capability to enhance contrast between different elements by specific deuteration of a particular constituent of the material [8]. The unique capability of neutrons to probe magnetic materials through pure magnetic scattering events is also exploited to unravel novel magnetic behaviors and magnetic materials. These include the development of superconductor materials with higher transition temperatures, the study of topological matter or the characterization of novel alloys with advanced magnetic properties [9,10]. Finally, due to neutron being composed by three quarks, events such as collisions between neutrons, all of them traveling at relativistic speeds close to the speed of light, are used to get an insight on new physics beyond the Standard Model of particle physics [11].
Another perspective from which neutron science can be considered as an incomparable tool to study materials is within one of the main conceptual frameworks determining science policies nowadays: their contribution to properly address grand challenges of current society. Environmental challenges such as pollution can be tackled by neutron reflectometry and diffraction experiments, which determine the intrinsic nature of contaminants within the media they pollute [12]. Energy generation and storage, together with the transition to environmental friendlier materials and circular economy paradigms, are areas where neutrons can help [13]. Information and communication technologies are also improved thanks to neutron sciences, where spintronic devices, ferroelectric materials, novel magnetic data storage material candidates and sensors or actuators, among others, are being intensively studied with neutrons [14]. The unique capabilities of neutrons as a non-invasive deeply penetrating imaging tool to reveal structures in real time, allows obtaining 3D images of inner parts of different cultural heritage materials [15]. Further, neutron scattering experiments are being essential to study degenerative diseases such as Alzheimer, targeted drug delivery systems or dental and bone implants, among others [16].
As an excellence center aiming to develop “new materials for a better life”, BCMaterials, Basque Center for Materials, Applications and Nanostructures, is devoted to the development of a new generation of materials contributing to advanced and sustainable technologies. In this scope, neutron sciences are an essential tool in our understanding on materials, guiding both materials design and applications. The international effort that represents science has allowed our researchers to successfully perform neutron scattering experiments in different neutron sources around the world, including the Institute Laue Langevin (ILL) in Grenoble, France; the ISIS neutron and muon source in Oxfordshire, UK; the FRM-II in Munich, Germany; the Budapest Neutron Center in Hungary; the IBR-II of the Joint Institute of Nuclear Research in Dubna, Russia; or the Spallation Neutron Source, part of the Oak Ridge National Laboratory in the USA, among others.
Five specific examples of scientific experiments performed by our researchers using neutron scattering techniques are presented hereafter. The first example, in the area of materials for energy applications, consists in a combination of neutron diffraction, NR and SANS experiments, to investigate a new generation of organic solar cells with enhanced durability, essential for the new energy generation paradigms. More concretely, the degradation mechanisms of different perovskite-based organic solar cells are being currently studied through a combination of three different neutron scattering techniques: neutron reflectometry, small angle neutron scattering and neutron diffraction. The second example corresponds to the study of advanced multifunctional materials such as ferromagnetic shape memory alloys, which are magnetic materials that deform up to 15% upon the application of a magnetic field, and that are being studied by combining different types of neutron diffraction techniques, namely powder, single crystal and polarized single crystal neutron diffraction. The atomic site occupancies and magnetic spin densities are being analyzed in these materials, being these neutron experiments combined also with synchrotron X-Ray absorption measurements performed in SPring-8, Japan (Figure 3).
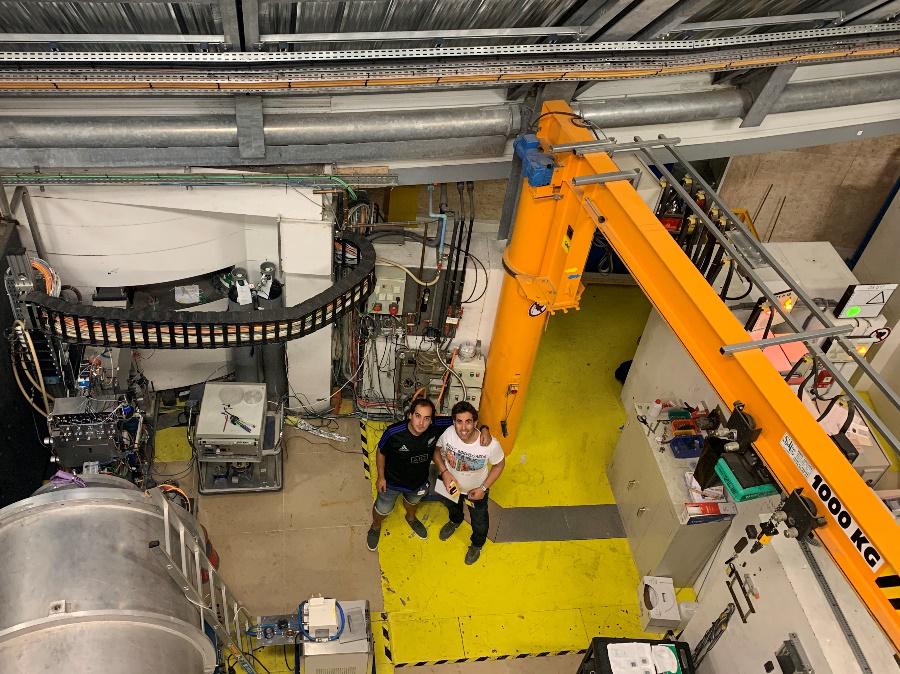
.
A third example is found in the study of nanostructured materials for biomedicine by means of SANS experiments, aiming to reveal the structure of magnetosome chains embedded in magnetotactic bacteria, a study aiming at improving cancer treatment by magnetic hyperthermia. The fourth example corresponds to materials envisaged to tackle environmental issues such as water remediation and purification from heavy elements, investigating different metal adsorption and functionalization processes of Metal-Organic Framework (MOF) materials, by means of inelastic and quasi-elastic neutron scattering experiments. Finally, the last example of neutron experiments performed by scientists at BCMaterials is found in the area of advanced manufacturing and technologies, where novel rare earth-free permanent magnets or materials for sensors and actuators are being investigated by means of neutron diffraction experiments.
Thus, in the complex challenge of developing advanced and multifunctional materials for advanced technologies allowing to tackle the grand challenges facing nowadays society, with respect to energy, environment, biomedicine or communication and information technologies, among others, neutron science appear as an essential tool to reach, sooner than later, a new generation of “materials for a better life”.
Acknowledgements
Basque Government Industry and Education Department under the ELKARTEK, HAZITEK and PIBA (PIBA-2018-06) programs, respectively.
References
[1] C. g. Brockhouse, Bertram N. ; Schull, The Nobel Prize in Physics 1994, (n.d.). https://www.nobelprize.org/prizes/physics/1994/summary/.
[2] T. Brückel, Forschung mit Neutronen in Deutschland – Status und Perspektiven, (2005). http://www.neutronenforschung.de.
[3] B.T.M. Willis, C.J.Carlile, Experimental Neutron Scattering, Anim. Genet. 39 (2009) 561–563.
[4] ESS project reports 2003 and update 2004, n.d. http://neutron.neutron-eu.net/n_documentation/n_reports/n_ess_reports_and_more.
[5] W. Doster, S. Cusack, W. Petry, Dynamical transition of myoglobin revealed by inelastic neutron scattering, Nature. 337 (1989) 754–756. https://doi.org/10.1038/337754a0.
[6] D.A. Neumann, J.R.D. Copley, R.L. Cappelletti, W.A. Kamitakahara, R.M. Lindstrom, K.M. Creegan, D.M. Cox, W.J. Romanow, N. Coustel, J.P. McCauley, N.C. Maliszewskyj, J.E. Fischer, A.B. Smith, Coherent quasielastic neutron scattering study of the rotational dynamics of C60 in the orientationally disordered phase, Phys. Rev. Lett. 67 (1991) 3808–3811. https://doi.org/10.1103/PhysRevLett.67.3808.
[7] M. (Universite des S. et des T. de L.F.A. (France). L. de D. des C.M. Bée, Quasielastic Neutron Scattering, 1988.
[8] F. Shu, V. Ramakrishnan, B.P. Schoenborn, Enhanced visibility of hydrogen atoms by neutron crystallography on fully deuterated myoglobin, Proc. Natl. Acad. Sci. U. S. A. 97 (2000) 3872–3877. https://doi.org/10.1073/pnas.060024697.
[9] Q. Huang, Y. Qiu, W. Bao, M.A. Green, J.W. Lynn, Y.C. Gasparovic, T. Wu, G. Wu, X.H. Chen, Neutron-diffraction measurements of magnetic order and a structural transition in the parent BaFe2As2 compound of FeAs-based high-temperature superconductors, Phys. Rev. Lett. 101 (2008). https://doi.org/10.1103/PhysRevLett.101.257003.
[10] F. Katmis, V. Lauter, F.S. Nogueira, B.A. Assaf, M.E. Jamer, P. Wei, B. Satpati, J.W. Freeland, I. Eremin, D. Heiman, P. Jarillo-Herrero, J.S. Moodera, A higherature ferromagnetic topological insulating phase by proximity coupling, Nature. 533 (2016) 513–516. https://doi.org/10.1038/nature17635.
[11] D. Dubbers, M.G. Schmidt, The neutron and its role in cosmology and particle physics, Rev. Mod. Phys. 83 (2011). https://doi.org/10.1103/RevModPhys.83.1111.
[12] G.J. Cuello, G. Román-Ross, A. Fernández-Martínez, O. Sobolev, L. Charlet, N.T. Skipper, Pollutant Speciation in Water and Related Environmental Treatment Issues, in: 2009: pp. 491–520. https://doi.org/10.1007/978-0-387-09416-8_17.
[13] F.M. Mulder, T.J. Dingemans, H.G. Schimmel, A.J. Ramirez-Cuesta, G.J. Kearley, Hydrogen adsorption strength and sites in the metal organic framework MOF5: Comparing experiment and model calculations, Chem. Phys. 351 (2008) 72–76. https://doi.org/10.1016/j.chemphys.2008.03.034.
[14] M.L. Baker, T. Guidi, S. Carretta, J. Ollivier, H. Mutka, H.U. Güdel, G.A. Timco, E.J.L. Mcinnes, G. Amoretti, R.E.P. Winpenny, P. Santini, Spin dynamics of molecular nanomagnets unravelled at atomic scale by four-dimensional inelastic neutron scattering, Nat. Phys. 8 (2012) 906–911. https://doi.org/10.1038/nphys2431.
[15] F. Casali, M. Bettuzzi, R. Brancaccio, M.P. Morigi, New X-ray digital radiography and computed tomography for cultural heritage, in: Sci. Cult. Herit. Technol. Innov. Case Stud. Mar. L. Archaeol. Adriat. Reg. Inl. Veli Losinj, Croat. 28 – 31 August 2007, 2010: pp. 85–99. https://doi.org/10.1142/9789814307079_0008.
[16] W. Yong, A. Lomakin, M.D. Kirkitadze, D.B. Teplow, S.H. Chen, G.B. Benedek, Structure determination of micelle-like intermediates in amyloid β-protein fibril assembly by using small angle neutron scattering, Proc. Natl. Acad. Sci. U. S. A. 99 (2002) 150–154. https://doi.org/10.1073/pnas.012584899.
Authors:
Jose Maria Porro1,2, Ainara Valverde1, Viktor Petrenko1,2, S. Lanceros-Mendez1,2
1BCMaterials, Basque Center for Materials, Applications and Nanostructures, PV/EHU Science Park, 48940 Leioa, Spain
2IKERBASQUE, Basque Foundation for Science, 48013 Bilbao, Spain
1 comment
This is a beautiful post. Congrats!.