Highly sensitive holographic polymers
Nowadays, there is a growing demand for new biosensors capable of detecting specific molecules, contaminants or toxic substances in food, drinking water, air, blood and so forth, with a high degree of sensitivity and specificity. Furthermore, we preferably want these biosensors to be inexpensive, easily reproducible and with a rapid and accurate response. This demand has led to the development of new strategies and processes in order to produce these sophisticated chemical sensing materials.
Biosensors are analytical devices based on a biological sensing element. As mentioned before, they are attracting an increasing interest due to the wide range of applications in which they can be used. To name a few, they can really mark a turning point in environmental analysis, clinical diagnostics, food safety or drug discovery 1. A biosensor consists of a recognition element and a signal transducer, the latter converting a physico-chemical change into a detectable response in real-time. Biomacromolecules based on proteins have been widely used as recognition element, but they have an important drawback: their restricted operating conditions, which makes their use quite complex in certain environments. That’s why there is a need for alternative receptors capable of responding to the presence of a target analyte with a high degree of specificity.
It seems that molecularly imprinted polymers (MIPs) are very promising as tailor-made, synthetic biosensors. A molecularly imprinted polymer (MIP) is a polymer whose polymerization has been carried out in the presence of a template molecule, as shown in figure 1. Once the polymerization has taken place, the template molecule is removed and a cavity remains instead, making the imprinted polymer complementary to the selected molecule. This process will allow the imprinted polymer to bind specific target analytes, thus providing an accurate mechanism of recognition.
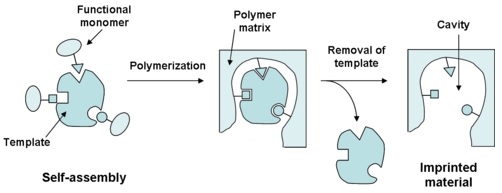
These molecularly imprinted polymers present good physical and chemical properties and they are already in use in several fields as recognition elements. Nevertheless, apart from the recognition element, a biosensor also needs a transducer in order to translate the recognition effect into an easy-to-use response. Optical mechanisms have proved particularly suitable in polymers, creating a response in the form of a pattern (photopatterning), thus building the transduction mechanism in the polymer itself. The recognition of the target molecule results in a change of the optical properties of the polymer, leading to a direct read-out in real-time.
An added advantage of these optochemical biosensors is that they can be processed in one step, creating the polymer and the pattern by photopolymerization. However, this single-step process is not that easy for molecularly imprinted polymers due to the considerable amount of different components that are needed in MIP’s polymerization: template molecules, monomer precursors, cross-linkers, photoinitiators, solvents, etc.
In a recently published article, the first example of a molecularly imprinted polymer film patterned with a hologram during photopolymerization has been reported. The result is a new functional holographic polymer designed to detect specific analytes. The study has been carried out by the Professor Haupt research group, from the Compiegne University of Technology in France 2. They have managed to create the molecularly imprinted polymer and the transducer mechanism in a single step through photopolymerization with interfering laser beams. In this study, the target analyte was the steroid testoterone, whose detection is quite interesting in fields such as sports and medicine.
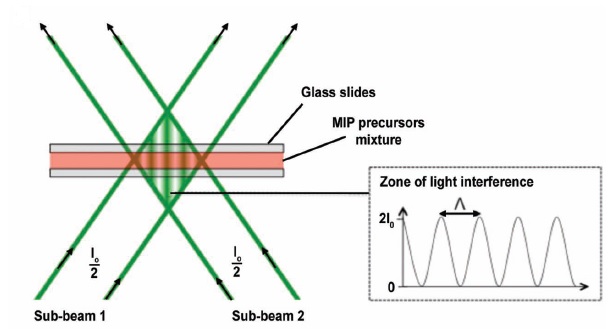
In this process, the optical pattern is generated during the polymerization of the precursor mixture by interference of two coherent laser beams, as shown in figure 2. The resulting sinusoidal light distribution induces an inhomogeneous photopolymerization, where the reactive molecules diffuse from dark to bright regions, in which they are consumed. This leads to a polymer film with different matter densities and with a diffraction grating, that is, a hologram.
Of course, the experimental procedure requires fine adjustments for optimal performance. A high spatial coherence of the laser beams is needed to obtain high contrast in the diffraction grating. Moreover, a slow polymerization is preferred because it allows the reactive species to properly diffuse and thus improve the holographic structure, so the choice of the photoinitiator is of utmost importance. Irradiation time, laser wavelength and intensity are also important parameters to be taken into account.
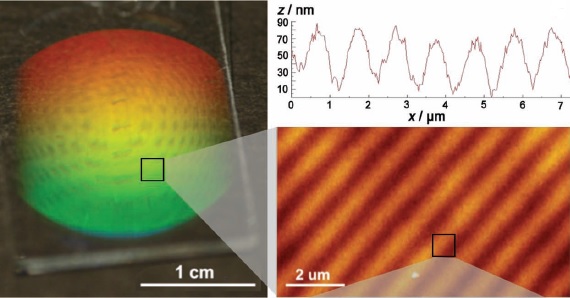
All these things considered, we should remember now that we were looking for a polymer capable of binding a target molecule and responding with an optical change. This optical response will be based on the diffraction efficiency of the polymer film, i.e., the ratio between the power diffracted into a particular order (zero-order, P(0); first-order, P(1);…) and the incident power (Pinc), as it can be seen in the scheme of figure 4. The diffraction efficiency is expected to change if the refractive index contrast between the polymer and the surrounding medium changes.
The results of this study have proved that when the polymer film is exposed to the target analyte, in this case the testosterone, a change in the refractive index of the polymer film takes place, thus confirming the molecular imprinting effect. Besides this, the monitoring of the diffraction efficiency confirms the presence of the target analyte, and this response will be different and specific for each molecularly imprinted polymer.
The development and optimization of this technique seem to be very promising in different fields where the detection of specific molecules is crucial. On the other hand, the way in which molecular imprinting and hologram writing are achieved in a single-step process can make the scalability of the process possible, thus allowing the fabrication of highly accurate, reproducible and inexpensive biosensors. These new polymers are likely to have a great impact on several fields where chemical sensing is required.
References
- Turner A. P. F., Biosensors: sense and sensibility, Chem. Soc. Rev., 2013, 42, 3184-3196. doi: 10.1039/c3cs35528d. ↩
- Fuchs Y., Soppera O., Mayes A., Haupt K., Holographic Molecularly Imprinted Polymers for Label-Free Chemical Sensing, Advanced Materials 2013, 25, 566-570. doi: 10.1002/adma.201203204. ↩
1 comment
[…] Rafael Barzanallana: Informática Magufos Partículas Biología Sort Share mappingignorance.org 1 minute […]