Quantum mechanics in biological systems (III): Magnetoception
Magnetoception, the fantastic ability to perceive magnetic fields. A skill though impossible for long. It was difficult to assume that a 0.5 Gauss Earth’s magnetic field (your fridge has one with 100 Gauss) could have some effect on living things. However, the magnetic field perception was supported since the very beginning by experimental observation and evidence: some animals are affected in their orientation or navigational behaviour by magnetic field variations. Some of those animals have an “internal compass”, helping them to keep their North-South orientation. Few of them are able of even more: to use Earth´s magnetic field as a GPS. They get positional information by previously learned destination field´s features and surrounding magnetic topography. It is said they have “magnetic maps”.
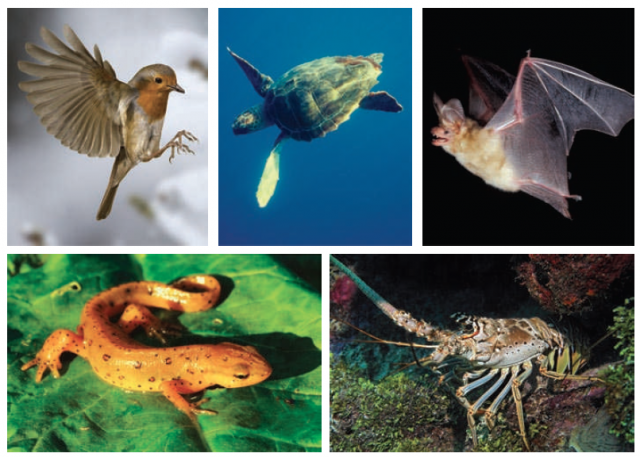
It is known that many animals use the stars, the sun positions, light polarization and color gradients to navigate, but seem to be obvious that to use magnetic-field sensing is far more robust and reliable if you are unable to build some kind of artificial map. Otherwise, how the migratory birds would be able to find their way, accurately, year after year even in cloudy dark days and nights. However, let´s to think for a second, we have the consequence but, what is the cause? Until not long ago, to find it was a challenge just for one thing: the living matter is transparent to magnetism. How to perceive a sort of field which is able to pass though a row of elephants unfazed? What kind of receptor might be able to sense and amplify something as weak as Earth’s magnetic field? The answer to those questions begins with a microscopic bacteria and it has still no ending but lots of direct or indirect quantum mechanics behind.
Salvatore Belline discovered the bacterial magnetotaxis in the early 60s, in the middle 70s Richard Blakemore 1 describes “novel structured particles, rich in iron, within intracytoplasmic membrane vesicles” (called latter ferromagnetic crystals within magnetosomes). He himself proposes the mechanism: these particles confer to the bacteria a magnetic moment placing them in the direction of the magnetic field. In many cases the advantage for them is not to know were is the north but to know were is up (oxygenated water) or down (water poor in oxygen and muddy bottoms). Then, they will choose what they like. Thus, magnetosome chains or arrays were like a passive system for those bacteria, exactly like the needle of a compass. In fact, bacteria were able to feel the weak Earth´s magnetic-field using this ferromagnetic structures even being dead.
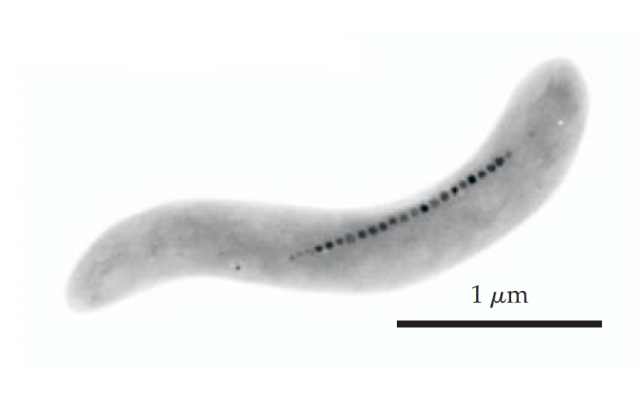
Afterwards, magnetite crystals were found gradually in other microscopic species, fungi and big animals that were previously assumed to have magnetoception capability. But in the latter cases the sole presence of a microscopic magnetite particles (F3O4) or other magnetic minerals in few cells of the body can not explain the orientation of the whole organism by that system. The torque generated by few picograms of magnetite can not alienate over a meter long and two hundred kilos loggerhead sea turtle with the magnetic field. The simplest scenario to explain how those magnetic structures work animals would be that the crystals may transmit its oscillation under the magnetic field by a sort of molecular mechanisms: Opening ion channels, generating conformational changes in different types of receptors, etc. The real scenario, though, is usually more complicated, and the molecular mechanism is still unknown, but the discovery of iron-mineral-based sensors and magnetite crystals located in the upper beak of some migratory birds point in that direction (Figure 3). We have further evidence of this magnetite-based magnetoception in migratory birds (in European robins in this case) was shown by Heyers et. al. PNAS 2010 2 Here it is shown that magnetic field variations leads to significant changes in neuronal activation in brain areas connected via trigeminal nerve with the upper break.
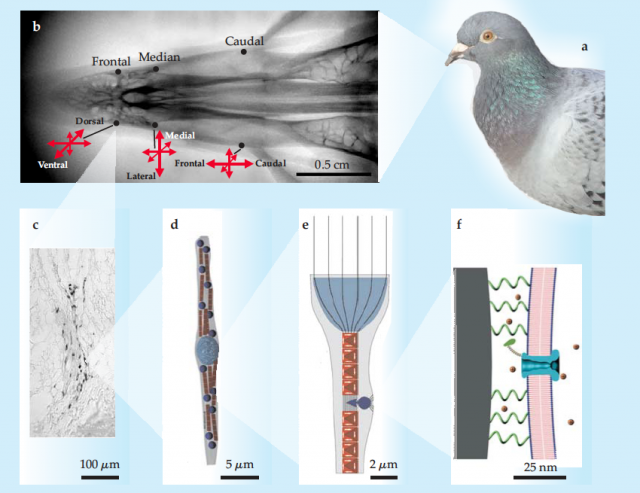
By now, the reader will not neglect that magnetism is an effect of quantum mechanics. The magnetic behaviour of materials at the atomic level is quite complex but, in short, one might say that the electron is a tiny magnet. It is a rotating electrically charged particle. In most materials, electrons are distributed randomly and their spin have different directions cancelling a possible resultant magnetic moment. In permanent magnets, because of its crystal structure (with a particular single-domain crystal size), electrons tend to be oriented so that the resultant of their magnetic moments generates a net magnetic force, large or small but noticeable. When a fragment of magnetic material is nanometric, it appears “superparamagnetism”, where the direction of magnetization randomly flip influenced by temperature. In the latter case, an external magnetic field could be able of magnetize the nanoparticles generating temporary magnets.
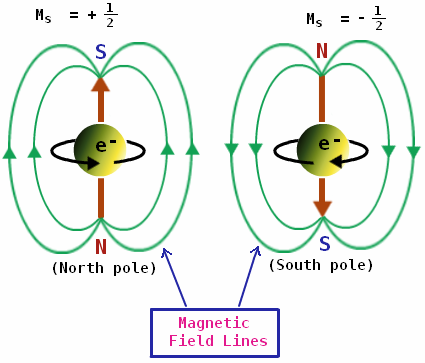
Therefore, here we have the first, though, indirect quantum mechanic effect over the ability of some living beings to perceive magnetic fields. The magnetic crystals exhibit a wide range of shapes and magnetic features. They are often different in different species. Some of them are single-domain crystals (0,1-1 um), meaning that they are permanent magnetos competent to align perse with the Earth’s magnetic field. These putative alignment would be the lever, the torque, which activates the receptor mechanism. Others are smaller than single-domain size and have different “superparamagnetic” properties. At body temperature they lack of a permanent magnetic moment loosing its torque. Nevertheless, under Earth magnetic fields they are just able to attract or repel other neighbour crystals, thereby deforming the matrix containing the crystal array. Then probably, different magnetic phenomena arising from some magnetic materials underlay the mechanical molecular mechanisms acting as magnetic sensor.
But that’s not all folks, the above putative magnetoception mechanism seems not to be alone, at least, not to guide some migratory birds in their flight. Avian retina could hide another possible mechanism 34 able to feel the Earth´s magnetic-field: a chemical, light-dependent, radical-pair-based magnetoreception. The first clues about it emerged because the orientation of certain migratory birds was modulated by the presence of specific light wavelengths. Several candidates among the light sensing molecules found in the eye of birds have been considered as molecular sensors for magnetism. The most appropriate candidates seem to be the cryptochromes. They are proteins able to absorb light leading to photoreduction processes by transfer of an electron to a molecule called a chromophore. The transference is mediated by some tryptophan residues in cryptochrome´s structure, being here FAD (flavin adenine dinucleotide) the named chromophore. Cryptochromes have been found also in the eyes of drosophila and committed to their behaviour under magnetic fields. In plans, e.g. Arabidopsis cryptochromes are well known, they involve in photosensitivity and circadian rhythms control, being the reduction state of the FAD molecule the reporter of the status light vs no light (Figure 5). As a part of the electron-transfer process, radical pairs are formed (FADH + Trp400+, FADH + Trp377+, FADH + Trp324+) as well as oxygen radicals. These radical pairs are known to be sensible to magnetic fields.

Here we find what is this to do with quantum mechanics. Basically, those electron transference processes are highly depend on electrons spin relative orientations. The spins continuously change in the orientation of the rotational axis (precession), here a external magnetic field can work increasing the time that those electrons spend in a competent orientation to complete the transference. Therefore, the magnetic field could avoid that an unpaired electron on FADH transfers back to one of those tryptophans, maximicing the time of cryptochrome activation. Although it remains unclear whether these radical pairs or oxygen radicals are responsible for the magnetocepcion and the molecular mechanisn is only a theory, the output is fancied as cryptochrome´s magnetic field readout overlaying on visual field of a bird (Figure 5).
About the magnetocepcion there are variety of conflicting views and some colliding when not excluding evidences. The last magnetoception mechanism is still highly controversial, but if finally it holds true, it will be another example of direct quantum effect used by living beings in their benefit. In the other side, ethological and physiological experiments leave no doubt, the magnetocepcion exists and, at least, in birds seems to have two systems involved (chemical, light-dependent, radical-pair-based and trigeminally mediated, iron-mineral-based). However, it is a challenge for the future to observe in vivo whether the proposed molecular mechanisms are necessary and sufficient to capture the weak Earth´s magnetic field and how they work at atomic level. Fascinating will be the time we discover how the live is able to feel something so ethereal and inexistent to our senses.
References
- Blakemore, R. Magnetotactic Bacteria. Science 190, 377–379 (1975) ↩
- Heyers D., Zapka M., Hoffmeister M., Wild J.M. & Mouritsen H. (2010). Magnetic field changes activate the trigeminal brainstem complex in a migratory bird, Proceedings of the National Academy of Sciences, 107 (20) 9394-9399. DOI: 10.1073/pnas.0907068107 ↩
- Rodgers, C. T. & Hore, P. J. Chemical magnetoreception in birds: The radical pair mechanism. Proc. Natl. Acad. Sci.106, 353–360 (2009) ↩
- Mouritsen, H. & Hore, P. The magnetic retina: light-dependent and trigeminal magnetoreception in migratory birds. Curr. Opin. Neurobiol.22, 343–352 (2012) ↩
2 comments
[…] mappingignorance.org […]
More biophysics please and thank u