Optics meets genetics to unravel the brain: the case of sex and aggression
You have probably heard this sentence: “the brain is the most complex organ in the universe”. Let´s dive inside such assertion to get a deeper feeling. The human brain contains around 86.000 million of neurons distributed across a bunch of brain areas (roughly 190). That´s one thing, not everything. Even more complex is how those neurons connect among them. In the cortex, each neuron makes synapses (connects) with near 10.000 other cells on average (see note below). Sometimes they contact nearby neurons (establishing local circuits), sometimes they send long-range projections to distant areas in the brain. Each brain area has a unique pattern of connectivity with specific target regions 1.
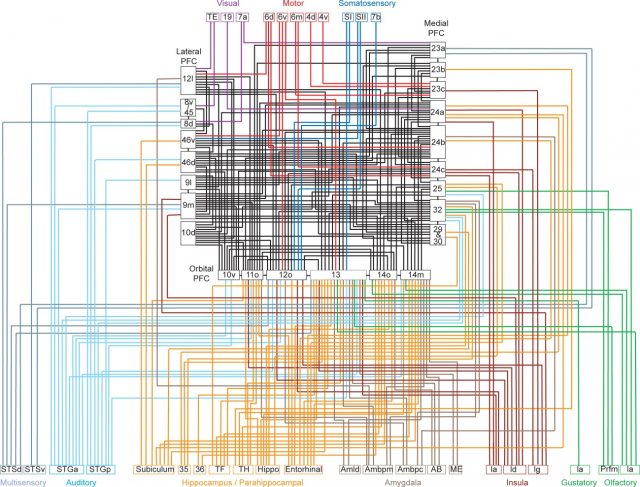
Even more. Overlapped to this, neurons make use of a certain neurotransmitter (sometimes more than one) to send messages to the other neurons. This chemical signal can exert different and opposing effects. May be excitatory, inhibitory or both depending on the receptors present in the target neuron. Glutamate, GABA, Serotonin, Dopamine, Noradrenalin and Acetylcholine are the most famous neurotransmitters/neuromodulators, existing from 1 to over 10 different types of receptors for each.
These levels (brain area/neurotransmitter/receptor) combine in a permutational manner to define an elementary unit of brain function: the neuronal type. Every brain area contains a variety of neuronal types which typically speak a different language (a unique firing pattern of nerve impulses) depending on their specific ion channels and the excitatory/inhibitory input they receive.
Even though, this definition of brain unit is not completely satisfactory. Pieces of neurons (individual synapses or dendritic compartments) can perform independent computations. Also, neuronal functioning is not static. It can change (what is known as neuronal plasticity) adapting to experience (which underlies rather diverse psychological phenomena such as learning or drug addiction).
Last but not least, whatever defines a neuronal type, inside each of them there is a specific repertoire of molecules that carry out every function wherein (firing pattern, intracellular signalling, metabolism, gene expression, etc.). Thus, understanding how the brain works (during a given process) means deciphering every single element at every single level: each molecule, within each cellular compartment or synapse, in each type of neuron and each one of its projections, in every nucleus or brain area in particular, and even at a higher scale, apprehending how all those diverse brain zones entangle a precisely timed symphony that gives rise to the variety of existing brain rhythms. Fortunately or not, those elements at each level are reutilised for a variety of purposes. There is no molecule exclusive to memory processes, for example, regardless of how concrete that memory would be. That single molecule will play a role in a bunch of memories and other brain functions as well. This constitutes a problem as fascinating as formidable.
Modern tools to deconstruct neural circuits
Neuroscientists have been struggling for a while to tackle the enigma of the brain and searching for appropriate methods to address its complexity. Interestingly, the recent years have seen the birth of a technology that is revolutionizing neuroscience. Its name is optogenetics (Nature´s method of the year in 2010). It reflects how the intersection of different scientific fields (here, solid state optics and molecular genetics) can result in a fertile territory that boosts qualitative steps forward in knowledge. Optogenetics consists on reengineering neurons to make them responsive to light 2, exploding a type of molecule called opsins (similar to the photoreceptors in our eyes that allows us to see). Since opsines are proteins, the DNA of the genes that encode them can be introduced in cells to achieve their presence in neurons. Opsins are varied and can drive either neuronal activation or silencing in response to light, making it possible to investigate what specific neuronal types do in concrete brain processes. Furthermore, it is permitting to address the role of specific projections, since it allows to manipulate (rather inhibit) a given connection without interfering with the global function of that neuron or its remaining connections. Other technologies have also been developed recently for a similar purpose, such as the pharmacogenetics approach which shows slower kinetics.
These techniques bear a clinical promise. Once unravelled the precise types of neurons and projections that are altered in animal models for neuropsychiatric diseases, it will be possible to analyse what molecules are changed within those specific locations. Thus, it might bring to reality the design of more precise pharmacological interventions than those currently available (many fail to work or elicit undesirable side effects). Parkinson, depression, anxiety and drug addiction are a few cases where optogenetic examination is underway. Furthermore, optogenetics is also allowing to clarify the circuits responsible for the effect of certain therapies such as deep brain stimulation or chemicals that are effective but which mechanism of action is not clearly understood. This is a second avenue by which optogenetics might prove helpful in hospitals in the long-term.
Optogenetics also has its limits. First, there are concerns inherent to animal models for neurological and psychiatric diseases. No model can recapitulate the full symptomatic complexity of human illnesses such as schizophrenia. But they can reproduce concrete aspects of them 3. Second, some traits of the architectural complexity of neuronal circuits could never be accessible. Single axons could branch and impinge on multiple target areas in a symptom-relevant manner, and/or they could deliver atypical cocktails of neurotransmitters that might be important for the symptomatic diversity of single diseases. The future will tell if optogenetics can be refined to meet these potentially important challenges.
The sexual and aggressive brain
Recent studies have exemplified the power of optogenetics to identify the role of a single neuronal type in behaviours that greatly shape our social life. Yes, they are sex and aggression. Both are practically ubiquitous in the animal kingdom. Like other survival functions, they depend on a brain region called Hypothalamus, a kind of air-traffic controller inside the brain that organizes behavioural responses to satisfy primary needs such as energy balance, physical integrity and reproduction.
A group of researchers leaded by David Anderson at CalTech (USA) have managed to activate optogenetically a specific neuronal type (containing the estrogen receptor 1 alpha, which mediates the effects of hormones that regulate the appearance of sex specific traits) within a particular nucleus inside the hypothalamus (the ventromedial hypothalamus) in male mice. As a result, the mice became super aggressive 4. They haphazardly attacked whatever it was near, whether a male, female or an inflated glove. Strikingly, when researchers stimulated neurons using low power light, the mice did not attack but started copulating with a nearby mouse (regardless the latter was female or male). When the same mouse was stimulated with high power light, it then started attacking to the very same partner it was mounting seconds before. With low power, light was recruiting a smaller fraction of neurons, suggesting that sex and aggression are encoded in this brain region by a population code (few neurons signal sexual drive, many neurons signal anger).
These studies show how optogenetics can elucidate the neuronal substrates of behaviour in the context of sex and violence. Perhaps, this knowledge might prove useful in future to allow interventions in cases in which violent and sexual drives are uncontrollable impulses.
Note:
In the cortex, the number of synapses is 1.5 x 10145 and the number of neurons is 19% of 8.6 x 10106, resulting in 9179 synapses/neuron
References
- Averbeck, B.B. and M. Seo, The statistical neuroanatomy of frontal networks in the macaque. PLoS Comput Biol, 2008. 4(4): p. e1000050. DOI: 10.1371/journal.pcbi.1000050 ↩
- Deisseroth, K., Circuit dynamics of adaptive and maladaptive behaviour. Nature, 2014. 505(7483): p. 309-17. PMCID:PMC406928 ↩
- Arguello, P.A. and J.A. Gogos, Modeling madness in mice: one piece at a time. Neuron, 2006. 52(1): p. 179-196. DOI:http://dx.doi.org/10.1016/j.neuron.2006.09.023 ↩
- Lee H., Ryan Remedios, Todd E. Anthony, Angela Chang, Linda Madisen, Hongkui Zeng & David J. Anderson (2014). Scalable control of mounting and attack by Esr1 neurons in the ventromedial hypothalamus, Nature, 509 (7502) 627-632. DOI: http://dx.doi.org/10.1038/nature13169 ↩
- Pakkenberg, B., et al., Aging and the human neocortex. Exp Gerontol, 2003. 38(1-2): p. 95-9. ↩
- Azevedo, F.A.C., et al., Equal numbers of neuronal and nonneuronal cells make the human brain an isometrically scaled-up primate brain. J Comp Neurol, 2009. 513(5): p. 532-41. ↩
2 comments
[…] leyendo en “Optics meets genetics to unravel the brain: the case of sex and aggression” (Mapping […]
The idea of deliberately putting ‘exploding’ proteins into brains is somewhat disturbing!