Harvesting heat to create electricity: A new world record
Approximately 90% of world’s electricity is generated in turbines moved by hot steam, which, unfortunately, operate only at 30 to 40 percent efficiency. This means that around two thirds of the total energy input is lost into the environment as waste heat. Thermoelectric materials can convert such waste heat into useful electricity, although they are not yet efficient enough to be applied industrially in a large scale. However, this might start to change as improving the efficiency of thermoelectric materials is targeted by many researchers worldwide. Big improvements are being made, among which the recent discovery by Li-Dong Zhao et al. that SnSe solid is the most efficient thermoelectric known to date clearly stands out 1.
It is almost 200 years since the Estonian physicists Seebeck first realized that a circuit made of two unlike metals with junctions at different temperatures deflected a compass. It was the first thermoelectric effect ever observed. Thermoelectric effects refer to both when a temperature gradient creates an electrical current or an electric potential creates a temperature difference. The applications of thermoelectrics are thus twofold: they can be used to generate electrical power from a temperature gradient or as refrigerators when a voltage is applied.
Even if all materials show thermoelectric effects, semiconductors are the most appropriate materials for building thermoelectric devices. At the atomic scale, if a temperature gradient is applied to a semiconductor the charge carriers diffuse from the hot side to the cold side creating an electrical current. The opposite can also happen, if applying a voltage an electrical current is established in a semiconductor the side from which the carriers are drifting cools down. In order to enhance the number of charge carriers and make these effects stronger, semiconductors used for thermoelectric applications are doped. Combining n-doped semiconductors, where extra electrons have been injected, with p-doped semiconductors, where electrons have been removed and the majority carriers are holes with positive charge, thermoelectric devices for power generation and refrigeration can be engineered as shown in Figure 1.
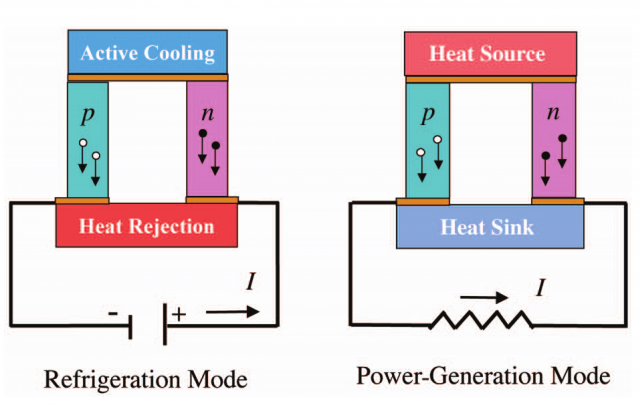
The low efficiency of thermoelectric semiconductors, however, has limited their application to situations where efficiency and cost are less important than reliability, for example in satellites and rovers exploring planets, like the Curiosity rover sent to Mars a few years ago (see Figure 2).
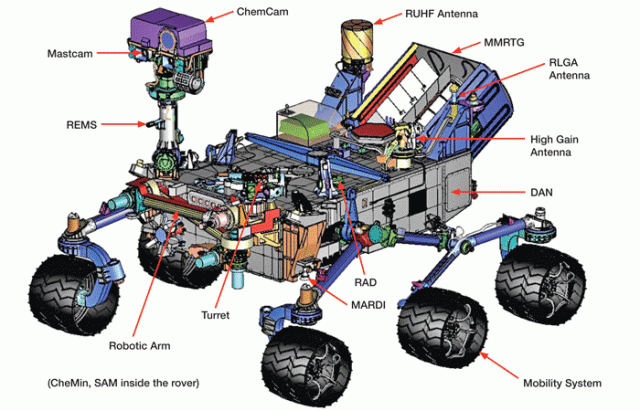
The efficiency of a thermoelectric material is determined by the dimensionless figure of merit (ZT) defined as
ZT = (S2σ/κ)T (1)
The higher ZT the more efficient the energy conversion and more electricity is recovered from heat. In equation (1) T is the temperature, S the Seebeck coefficient, which measures the voltage generated by the temperature gradient, σ is the electrical conductivity and κ the thermal conductivity. The interpretation of the equation is rather straightforward, good thermoelectric materials generate a large potential from the temperature gradient and conduct electrons well, while poorly conduct heat in order to prevent the dissipation of the temperature gradient. As some people say, we need an electron crystal but a phonon glass. Finding materials above the ZT > 2.5 value is supposed to have important implications for commercial deployment. As a reference, Curiosity rover’s thermoelectric generators are made of PbTe, whose ZT is around 1.4.
The ZT = 2.6 value that Li-Dong Zhao et al. have observed in SnSe crystals is, therefore, extraordinary and extremely promising. Even if at low temperature SnSe has a rather low thermoelectric efficiency, this rapidly soars at high temperature and reaches very high values above approximately 800 K (see Figure 3). According to these researchers from the Northwestern University and the University of Michigan in the USA, the origin of the high ZT values at high temperature is the ultralow thermal conductivity observed in the SnSe crystals. In fact, as shown in Figure 3, the enhancement of the figure of merit coincides with the suppression of the thermal conductivity. From equation (1) it is easy to understand why the suppression of κ, which appears in the denominator,makes ZT be highly increased.

The thermal conductivity has two different sources, the contribution of the electrons and the lattice vibrations. As expected for semiconductors, in SnSe the thermal conductivity is dominated by the lattice contribution. It is when the lattice thermal conductivity is suppressed that ZT soars. Indeed, the electronic contribution to the thermal conductivity and the electrical conductivity are related: the higher σ, the larger the electronic contribution to κ. That is why metals that conduct well electricity are good conductors of heat too. Consequently, it seems that discovering materials with very low lattice thermal conductivity is the most promising path to discover new efficient thermoelectrics. The new ZT record obtained by Li-Dong Zhao et al. is a clear fingerprint of the latter.
The suppression of the lattice thermal conductivity and the resulting enhancement of the thermoelectric figure of merit coincides with a displacive phase transition that SnSe undergoes at around 750 K. This raises the interesting question of whether the ultralow thermal conductivity of the high-temperature phase is intrinsic to it or it is induced by the proximity to the phase transition. Moreover, the authors also find that the large ZT only occurs within the planes of the SnSe layered crystal structure, specially because the electrical conductivity is lower in the out-of-plane direction. These remarks might motivate future research in this and similar systems.
The finding by Li-Dong Zhao and coworkers is an important step forward towards future commercial applications of thermoelectrics. Moreover, in contrast to PbTe and other lead based thermoeletrics, SnSe is composed of earth-abundant low-toxicity elements. The authors conclude their paper remarking that their work demonstrates that high ZT can be realized in simple systems without nanostructuring, which has previously been used to enhance the figure of merit in other thermoelectric materials 2. It seems we are in the right track to bringing the applications of thermoelectric devices from Mars to the Earth.
References
- Zhao L.D., Yongsheng Zhang, Hui Sun, Gangjian Tan, Ctirad Uher, C. Wolverton, Vinayak P. Dravid & Mercouri G. Kanatzidis (2014). Ultralow thermal conductivity and high thermoelectric figure of merit in SnSe crystals, Nature, 508 (7496) 373-377. DOI: http://dx.doi.org/10.1038/nature13184 ↩
- Kanishka Biswas, Jiaqing He, Ivan D. Blum, Chun-I Wu, Timothy P. Hogan, David N. Seidman, Vinayak P. Dravid, and Mercouri G. Kanatzidis, “High-performance bulk thermoelectrics with all-scale hierarchical architectures”, Nature 489, 414 (2012). ↩
1 comment
[…] [Continue reading at MappingIgnorance] […]