Novel sources of clean energy: Where functional polynitrogen materials meet society’s needs.
Author: Stefano Battaglia is a Ph.D. student (ITN-EJD-TCCM) at Université de Toulouse III
Since the Nobel Prize in Physics was awarded in 2010 to Andre Geim and Konstantin Novoselov for groundbreaking discoveries on the two-dimensional material graphene12, the research in low-dimensional carbon nanostructures has seen an incredible increase of interest. Among the different types of structures, carbon nanotubes (CNTs) (experimentally synthesized for the first time in 1991 by Sumia Iijima34) present a wide array of possible applications, spacing from electronics, through energy storage, to biology and medicine.
Carbon nanotubes consist in one (or more) graphene sheet(s) rolled and connected at the two opposite edges (Figure 1).
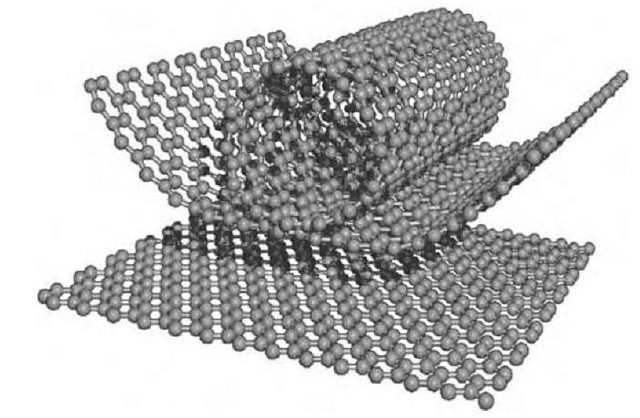
Depending on the way they are wrapped, different kinds of properties may arise, which eventually completely change their possible field of application. To identify the different types of CNTs, we conventionally use two numbers, n and m, with n always greater or equal to m. Carbon nanotubes can be classified into two categories: achiral nanotubes, which have either n equal m (armchair type) or m equal zero (zig-zag type), and chiral nanotubes which comprise all the other allowed combination of n and m, i.e. whit m different from zero and from n. Figure 2 illustrates the three typologies of nanotubes.
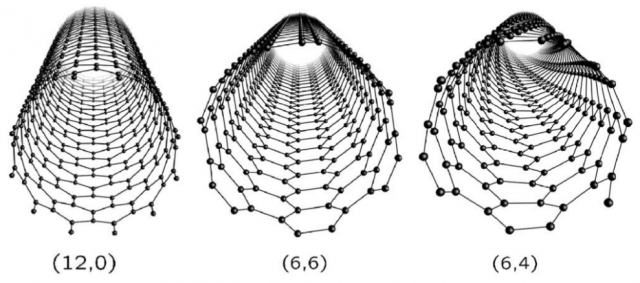
In recent years, CNTs have been proposed as suitable hosts for other molecules, either functioning as a catalyst for reactions happening within the tube or to confine certain types of compounds to decrease their reactivity. In particular, molecular confinement inside carbon nanotubes has been suggested as a possible way to stabilize highly energetic nitrogen chains56, which would otherwise quickly dissociate at ambient conditions.
Polynitrogen compounds are very promising systems for creating high energy-density materials (HEDMs) because of their exceptional energy difference between single, double and triple N-N bond7. The triple bond is incredibly stable and hard to break (229 kcal/mol), while the single bond is relatively weak (38.4 kcal/mol). This feature can be exploited by synthesizing weakly bonded (i.e. singly or doubly bonded) systems, such that if the dissociation reaction can be controlled, it would be possible to release the energy in a supervised fashion by breaking the bonds and converting the polynitrogen molecules into environmentally safe N2 molecules.
The major challenge so far has been the stabilization of such molecules at ambient temperature. Despite the experimental observation of N3, N4 and N5, both the neutral and the charged systems, theoretical calculations predicted a polymeric N8 crystal to be stable at ambient temperature8. However, most of the just cited systems have an incredibly small lifetime and this is considerably slowing down the progress in this field.
The two most promising species are the long known trinitrogen anion and the recently synthesized pentanitrogen cation. The usual approach to store energy in form of unstable compounds (such as the nitrogen case) or gases (e.g. molecular hydrogen), is to enclose them inside tanks continuously kept at low temperatures and/or high pressure. Although this is an effective approach, the are clear limitations in terms of transportation and energetic requirements to move the tanks and to keep them cold!
Alternatively, since N3– and N5+ are charged systems (and with opposite charge), there have been studies trying to see if it is possible to create a stable molecular salt composed by N3– and N5+, however this attempt did not lead to satisfactory results91011.
Recently, following the idea of Abou-Rachid et al., there has been an attempt to stabilize these two ions through molecular confinement inside zig-zag carbon nanotubes 12 (Figure 3).
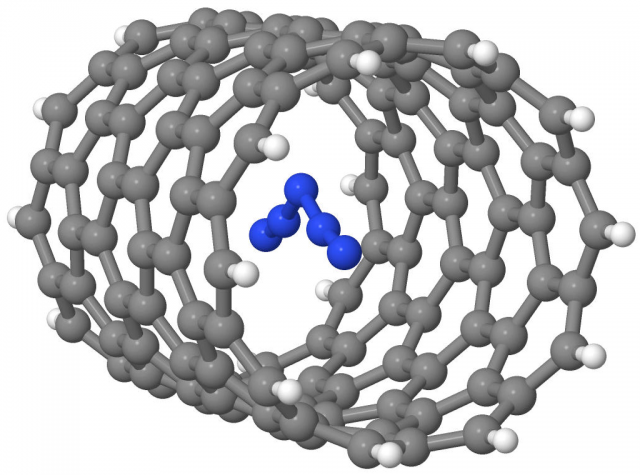
Theoretical calculations predict a favorable interaction between the host (CNT) and the guest (the two nitrogen ions), that is, the two molecules are stabilized if confined (independently) in the nanotube. According to the results, an optimal diameter for the nanotube has been found, which is of 6.26 Å to stabilize the N3– species and of 7.83 Å to stabilize the N5+ species. In the first case the unrelaxed interaction energy (i.e. without accounting for the effects of the confinement on the geometry of both the nanotube and the polynitrogen compound) has been estimated to be -1.3 eV, while in the second case it amounts to -1.67 eV. These two values are encouraging, since they fall right in-between the energy of a usual covalent bond (2 to 4 eV) and that of a purely non-covalent interaction (meV range). Between 0.1 eV and 1 eV is in general considered as a suitable energy range to optimally stabilize the molecules for energy storage purposes (i.e. there is no need for an extreme amount of energy to release them, but at the same time they are stable enough to “resist” small perturbations without triggering any unwanted release). There are still many open questions on this topic, such as which is the nature of the stabilization, which are the geometric and the electronic effects of the nanotube on the nitrogen compound, what happens if more ions are confined in the same tube, are ions oppositely charged stable if confined together, and many more.
To summarize, polynitrogen compounds are a promising type of material which has the potential to provide clean and inexpensive energy. Due to the incredible properties of nitrogen bonds, the amount of energy released is such that it could be employed as rocket fuels or jet propellers. The major challenge so far has been the difficult stabilization of such compounds at ambient conditions. To be able to get over this complication, more work is required from both the theoretical and the experimental communities, ideally with a jointly effort which would allow for a more rapid development of the field.
References
- Novoselov, K. S.; Geim, A. K.; Morozov, S. V.; Jiang, D.; Zhang, Y.; Dubonos, S. V.; Grigorieva, I. V.; Firsov, A. A. Electric Field Effect in Atomically Thin Carbon Films. Science. 2004, 306, 666–669. ↩
- Novoselov, K. S.; Geim, a K.; Morozov, S. V; Jiang, D.; Katsnelson, M. I.; Grigorieva, I. V; Dubonos, S. V; Firsov, A.A. Two-Dimensional Gas of Massless Dirac Fermions in Graphene. Nature 2005, 438 (7065), 197–200. ↩
- Iijima, S. Helical Microtubules of Graphitic Carbon. Nature. 1991, pp 56–58. ↩
- Iijima, S.; Ichihashi, T. Single-Shell Carbon Nanotubes of 1-Nm Diameter. Nature 1993, 363 (6430), 603–605. ↩
- Abou-Rachid, H.; Hu, A.; Timoshevskii, V.; Song, Y.; Lussier, L.-S. Nanoscale High Energetic Materials: A Polymeric Nitrogen Chain N8 Confined inside a Carbon Nanotube. Phys. Rev. Lett. 2008, 100 (19), 196401. ↩
- Ji, W.; Timoshevskii, V.; Guo, H.; Abou-Rachid, H.; Lussier, L. Thermal Stability and Formation Barrier of a High-Energetic Material N 8 Polymer Nitrogen Encapsulated in (5,5) Carbon Nanotube. Appl. Phys. Lett. 2009, 95 (2), 14–17. ↩
- Zarko, V. E. Searching for Ways to Create Energetic Materials Based on Polynitrogen Compounds (Review). Combust. Explos. Shock Waves 2010, 46 (2), 121–131. ↩
- Hirshberg, B.; Gerber, R. B.; Krylov, A. I. Calculations Predict a Stable Molecular Crystal of N8. Nat. Chem. 2014, 6 (1), 52–56. ↩
- Evangelisti, S.; Leininger, T. Ionic Nitrogen Clusters. J. Mol. Struct. THEOCHEM 2003, 621 (1–2), 43–50. ↩
- Gagliardi, L.; Orlandi, G.; Evangelisti, S.; Roos, B. O. A Theoretical Study of the Nitrogen Clusters Formed from the Ions N3-, N5+, and N5-. J. Chem. Phys. 2001, 114 (24), 10733–10737. ↩
- Fau, S.; Bartlett, R. J. Possible Products of the End-on Addition of N-3(-) to N-5(+) and Their Stability. J. Phys. Chem. A 2001, 105 (16), 4096–4106. ↩
- Battaglia, S.; Faginas-Lago, N.; Evangelisti, S.; Leininger, T. In preparation. ↩