Electrochromic materials enable smarter biosensors
Wearable devices are attracting increasing attention. The most familiar today are smartwatches and smart bands. The sensors in these devices monitor vital signs such as our temperature, heart rate, and even blood oxygen, but are unable to address biochemical parameters. One of the main reasons is the relatively short life of chemical sensors and biosensors. Multifunctional materials provide ways to interface disposable chemical sensors to smart devices. This article describes how electrochromic materials can be used in the construction of enzyme-based biosensors that can easily be coupled to smart devices.
What is electrochromism?
Electrochromism consists in the change of optical properties, usually colour, with oxidation state (Mortimer et al., 2013). This is used, for instance, in the construction of dimming glass and mirrors, such as the windows of Boeing 787 Dreamliner, or particular anti-glare rearview mirrors. There are many electrochromic materials, both organic and inorganic; viologens (organic) are used in mirrors and tungsten oxide (inorganic) in smart glasses. A third fascinating example is Prussian blue, linked to art and science since its discovery. Prussian Blue is a deep-blue inorganic material featuring iron (II) and iron (III) bridged in a cubic structure by cyano groups. When both irons are in the (II) state, the material becomes transparent and is commonly known as Prussian White. Prussian Blue has found many uses since its discovery in 1704. It was first used as a colour pigment, and it has featured in prominent works of art such as Van Gogh’s Starry night and Hokusai’s Kanagawa’s wave, as shown in figure 1.
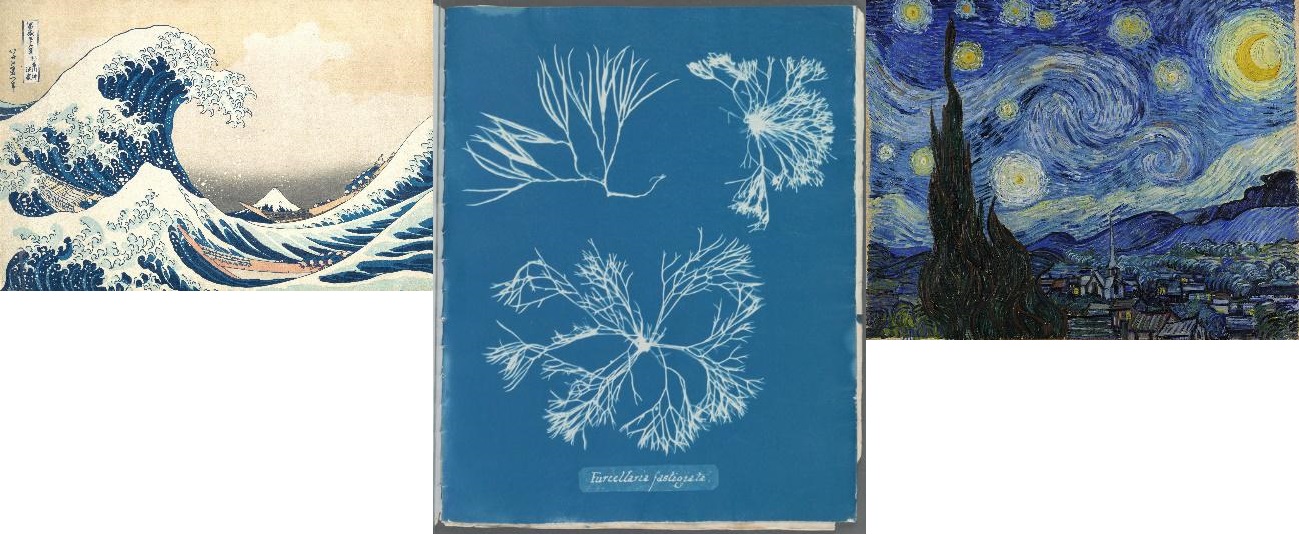
Prussian Blue was also a precursor of photography. The cyanotype process, also known as blueprinting, was invented by John Herschel in 1842. Anna Atkins, one of the first women scientists, used this process to make her beautiful and famous book on algae (Atkins, n.d.). Prussian Blue is also used in biology to spot the presence of iron in cells and tissues. More recently, Prussian Blue has found its way into biosensing (Karyakin, 2017). Prussian Blue catalyses the reduction of hydrogen peroxide, which is produced by oxidase enzymes and enables the construction of glucose, ethanol, lactate, and other biosensors.
Biosensor basics
A biosensor is an analytical tool designed to detect, quantify and sometimes even monitor a chemical or a biochemical species. According to its most conventional definition, depicted in Figure 2, a biosensor is “A device incorporating a biological sensing element either intimately connected to or integrated within a transducer” (“Biosensors and Bioelectronics,” n.d.). This definition contains two key elements. First, the recognition element in biosensors is of biological origin. The second essential feature of biosensors is that the recognition element must be bound to the sensor’s transducer. The transducer is the element in charge of converting the sensing event into an electrical signal
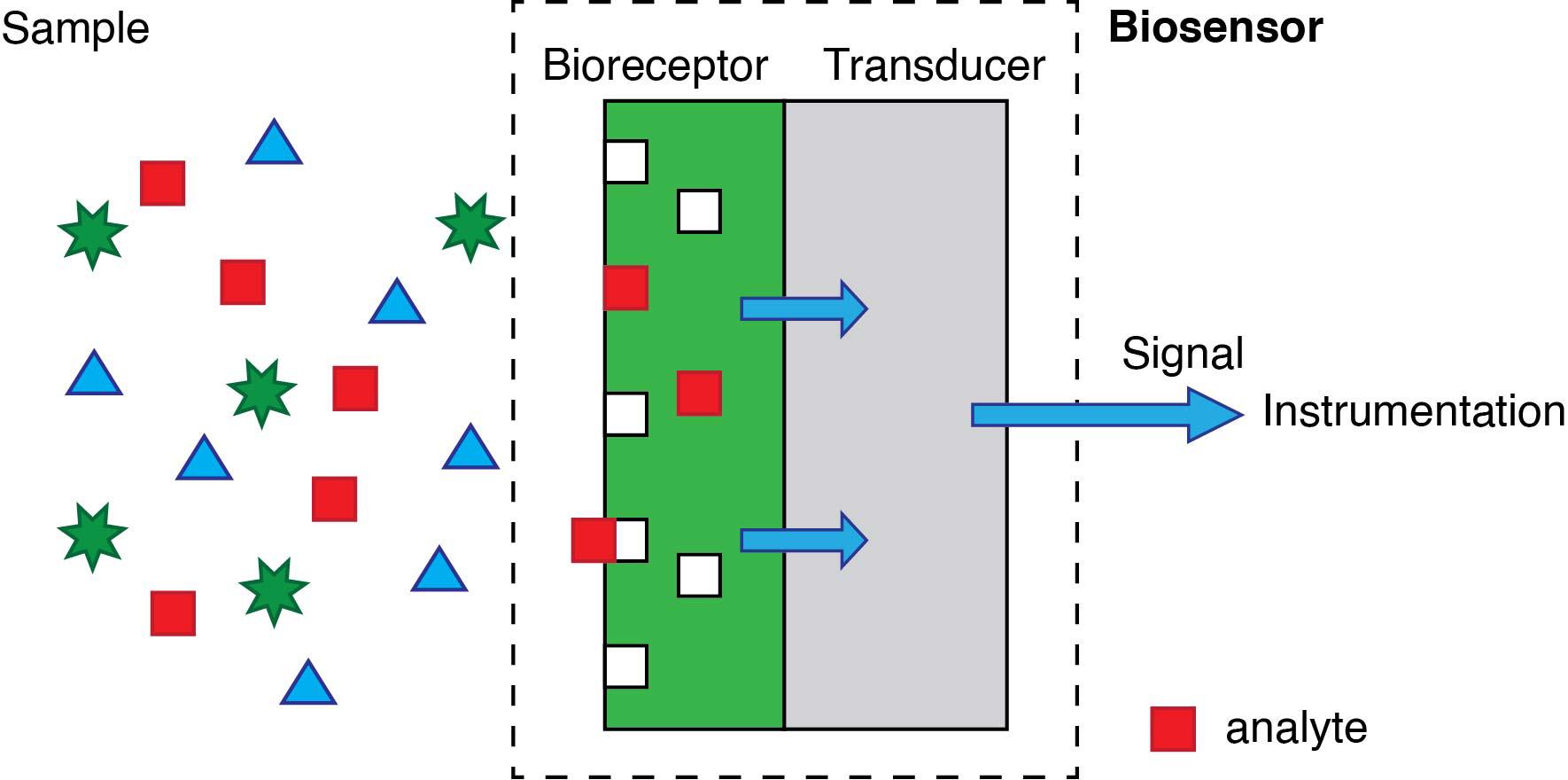
The biological recognition element can be an enzyme, an antibody, or a short sequence of genetic material. The primary advantage of using these -and other related- biomolecules is that they provide sensors with very high specificity. The ideal specific sensor will only produce a signal in the presence of the target analyte. However, most biological elements used -particularly enzymes and antibodies- will also display some affinity towards other molecules that are structurally (very) similar.
Regarding the transducer, it is usually an electrode or an optical component. Still, more recently, the surface of magnetic particles are also accepted. The “event”, on the other hand, is the biochemical process involving the analyte and the biorecognition element; it may be the activity of an enzyme on the target analyte, or the binding of an antibody to a target antigen, or the binding of complementary DNA base pairs arranged in a particular sequence.
Lyons and Clark reported the first glucose biosensor in 1962 (Clark and Lyons, 1962) . That first biosensor combined an oxygen electrode with glucose oxidase trapped in a dialysis membrane. The consumption of oxygen by glucose oxidase is proportional to the concentration of glucose present. Modern glucose biosensors have replaced oxygen by reversible redox species that shuttle electrons between the enzyme and the electrode (transducer) so that the current is proportional to the concentration of glucose (Wang, 2008).
Combining electrochromism and biosensing
Glucose biosensors are electronic devices involving two electrodes, but the processes taking part in the second electrode usually are not considered. This second electrode, or counter electrode, closes the circuit and its processes are not usually considered. However, suppose this electrode is made of an electrochromic material, such as Prussian Blue. In that case, it is possible to relate its colour change to the concentration of glucose (Aller Pellitero and del Campo, 2019). Such a system can work spontaneously in the presence of glucose, suppressing the need for external power sources. This is because the reduction potential of the Prussian Blue is higher than that of the biosensor electrode, resulting in a galvanic system. This construction combines three different functions in a single electrochemical cell: sensor, display, and power source. Besides, the system architecture and geometry can be designed so that the biosensor result can be read by sight or using a camera and software analysis (Pellitero et al., 2017).
A skin patch form factor: towards non-invasive measurements
Recently, a skin-patch for the determination of sweat lactate has been designed that is based on the above principle (Santiago-Malagón et al., 2020). The materials have been developed so that the biosensor can be screen printed on a thin conformal substrate. This skin-patch, shown in Figure 3, has a medical-grade adhesive layer to keep the biosensor in touch with the skin, while a Prussian Blue electrode is visible from the opposite side. Changes in the concentration of sweat lactate result in the conversion of Prussian blue to Prussian White over larger or smaller proportions of this display. Because the system is a single electrochemical cell, it does not require any connections or silicon-based components to function, which simplifies its construction enormously.
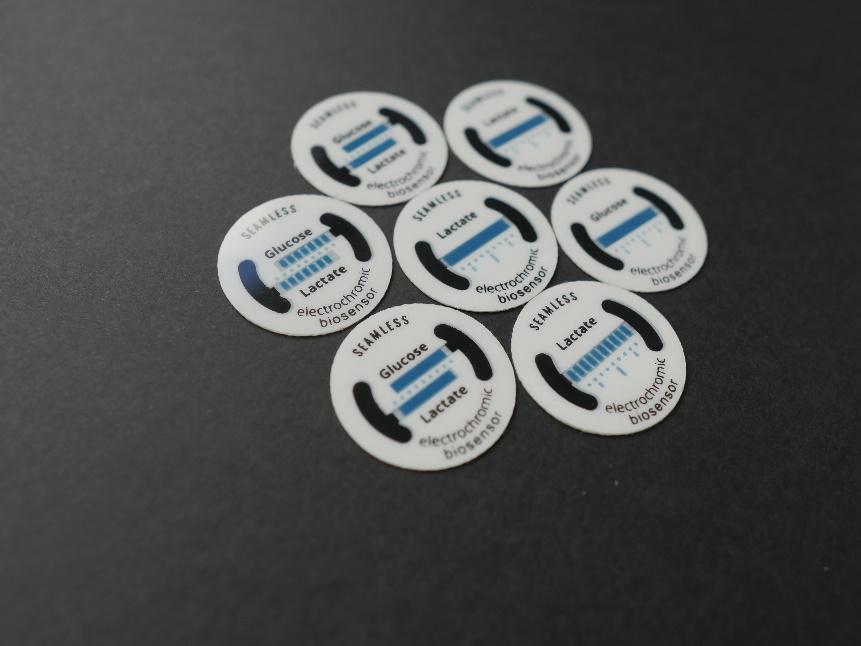
This is how it works. Lactate is always present in sweat. At rest, its concentration is in the low mM range, but it can reach as high as 50mM or more during exercise. The presence of lactate activates the biosensor which, in this configuration, is the cell anode. Electrons are relayed from lactate, which is oxidised, to the active site of the oxidase which, in turn, passes them to the metal centres of a special redox polymer. This redox polymer then passes the electrons to a graphite electrode that is connected to the transparent electrode containing a Prussian Blue coating. The Prussian blue is reduced by these electrons, changing its colour from blue to transparent. The two electrodes -biosensor and display- are positioned next to each other on the same plane. Because the electrons will flow through the path of least resistance, the Prussian blue closest to the biosensor is bleached first. Increasing the concentration of lactate increases both the cell voltage and the passing current. In other words, system power rises. This has significant consequences on the device operation. First, the colour change is faster (this is the effect of the higher current). Second, the display changes colour over a wider area. Since the display length is constant, this translates into longer distances away from the biosensor. How much longer will depend on the balance between cell voltage and internal resistance.
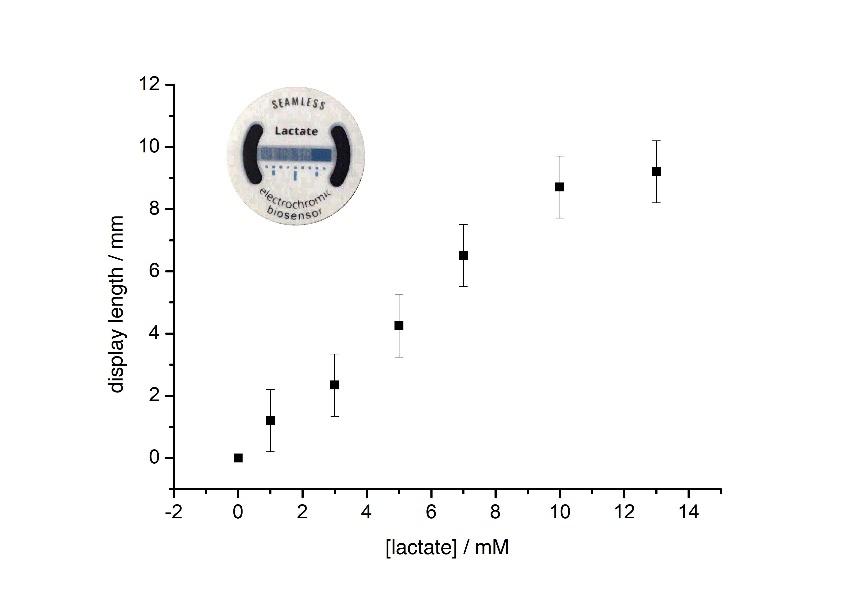
Final thoughts
Indeed, this proof of concept device has limitations. However, it shows how electrochromic materials can be exploited to produce efficient multifunctional components. In this case, the same electrochemical cell meets the functions of a sensor, power source, and information display. Moreover, the geometric arrangement of the electrodes enables the information to be easily interpreted by the user without the need for additional instrumentation. Higher levels of performance could be attained through small changes allowing the wireless -optical- interfacing with smart devices. The possibilities are endless, and the only limit is our imagination.
References
Aller Pellitero, M., del Campo, F.J., 2019. . Curr. Opin. Electrochem. 15, 66–72.
Atkins, A., n.d. Photographs of British Algae: cyanotype impressions.
Biosensors and Bioelectronics, Elsevier Journal
Clark, L.C., Lyons, C., 1962. . Ann. N. Y. Acad. Sci. 102, 29–45.
Karyakin, A.A., 2017. . Curr. Opin. Electrochem. 5, 92–98.
Mortimer, R.J., Rosseinsky, D.R., Monk, P.M.S., 2013. Electrochromic Materials and Devices, Electrochromic Materials and Devices. Wiley-VCH Verlag GmbH & Co. KGaA, Weinheim, Germany.
Pellitero, M.A., Guimerà, A., Kitsara, M., Villa, R., Rubio, C., Lakard, B., Doche, M.-L.L., Hihn, J.-Y.Y., Del Campo, F.J., Aller-Pellitero, M., Guimerà, A., Kitsara, M., Villa, R., Rubio, C., Lakard, B., Doche, M.-L.L., Hihn, J.-Y.Y., Javier del Campo, F., 2017. . Chem. Sci. 8, 1995–2002.
Santiago-Malagón, S., Río-Colín, D., Azizkhani, H., Aller-Pellitero, M., Guirado, G., del Campo, F.J., 2020. . Biosens. Bioelectron. 112879.
Wang, J., 2008. . Electrochem. Sensors, Biosens. their Biomed. Appl. 57–69.
Authors
Javier del Campo1,2 & Joseba Totoricaguena1
1BCMaterials, Basque Center for Materials, Applications and Nanostructures, UPV/EHU Science Park, 48940 Leioa, Spain
2IKERBASQUE, Basque Foundation for Science, 48013 Bilbao, Spain
1 comment
[…] Zenbait bulego modernotan kristal gardenak dira hormak, botoi bat sakatuta opaku bilakatzen direlarik. Leiho adimendun hauek material elektrokimikoa dute: tungsteno oxidoa. Material hauekin dabiltza lanean BC Materialsen biosentsoreak sortzeko: Electrochromic materials enable smarter biosensors […]