Dissipated heat into electrical energy using the thermoelectricity of concrete
Adopting a more sustainable energy portfolio is imperative to address and alleviate the impacts of climate change. A key process is energy harvesting versus energy “production”. In this vein, a potentially viable approach involves the conversion of dissipated heat into electrical energy via the use of thermoelectricity. This method has the potential to contribute significantly to our overall energy portfolio, provided there is a substantial enhancement in the thermoelectric efficiency of the materials.
The usefulness of a material in thermoelectric systems is determined by the device efficiency. This is determined by the material’s electrical conductivity (σ), thermal conductivity (κ), and the so-called Seebeck coefficient (S), which change with temperature (T). The maximum efficiency of the energy conversion process (for both power generation and cooling) at a given temperature point in the material is determined by the thermoelectric materials figure of merit, ZT = σS2T/ κ.
In order to enhance the energy harvesting capability of materials via thermoelectricity, it is necessary to optimize ZT. This can be accomplished by enhancing the Seebeck coefficient and electrical conductivity while simultaneously reducing the thermal conductivity. ZT of several materials has been enhanced to be above 2 at high temperatures, while the documented figure of merit of cementitious matrices falls within the range of 10−7 to 10−9. The investigation of concrete materials for the purpose of energy harvesting using thermoelectricity still necessitates a significant amount of research.
Several experimental studies have been conducted to enhance the thermoelectric properties of cement and concrete through the incorporation of various additives such as carbon fibers, silica fumes, intercalated carbon, metal oxides, steel fibers, carbon nanotubes, and graphene composites in optimized proportions. These composites facilitate the movement of electron and hole carriers, leading to improvements in the thermoelectric Seebeck coefficient and, consequently, in ZT. Still the highest known ZT for concrete materials, containing ZnO and graphene, is a mediocre 0.01.
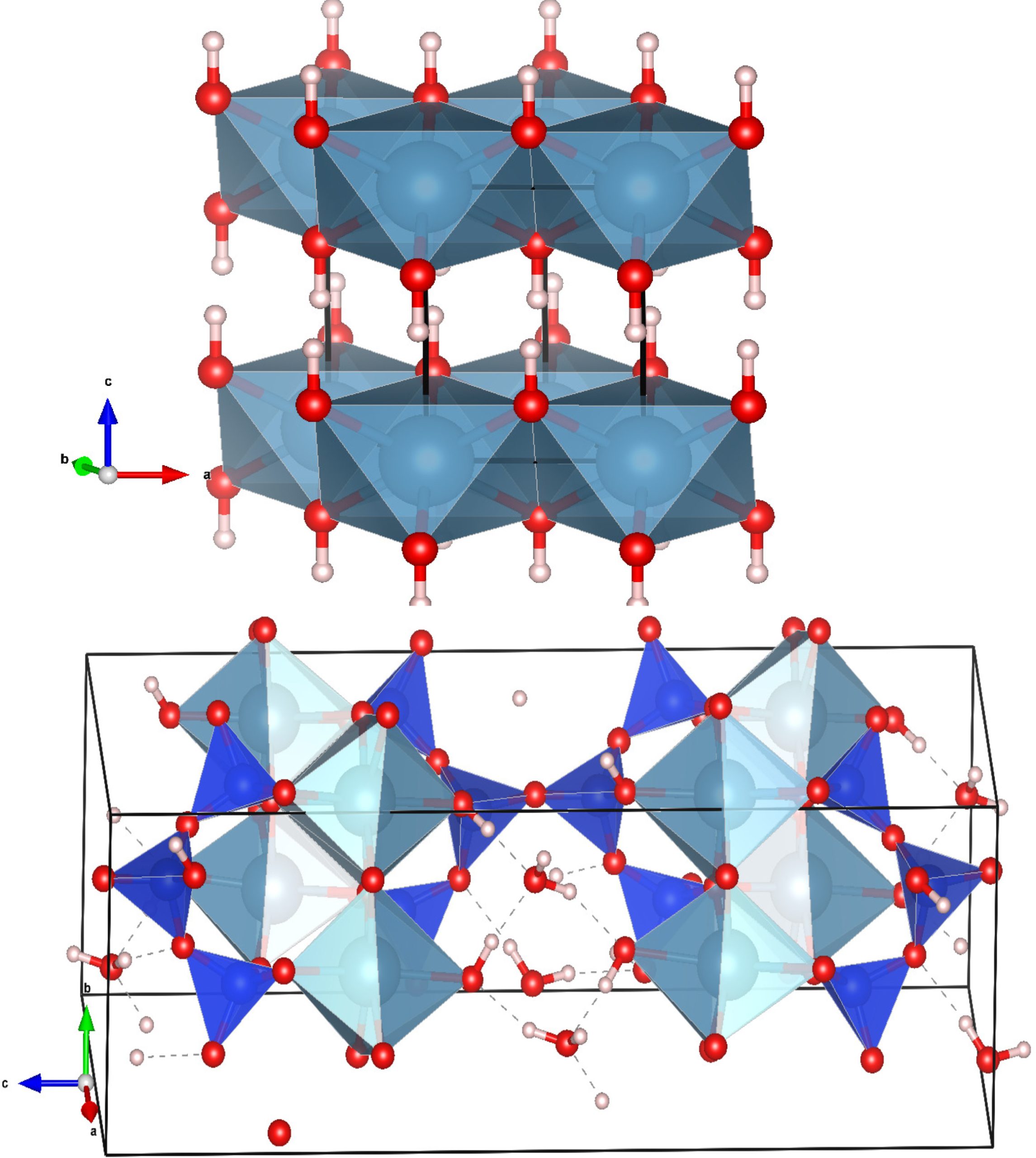
A cement is any of various substances used for bonding or setting to a hard material. A popular one, Portland cement, is a mixture of calcium silicates and aluminates made by heating limestone (CaCO3) with clay (that contains aluminosilicates) in a kiln. The product is then ground to a fine powder. When Portland cement is mixed with water, it sets in a few hours and then hardens over a longer period of time due to the formation of hydrated aluminates and silicates.
The hydration process is, thus, so important that some processes have been developed in order to activate cement, i.e., to enhance the early hydration rate. The hydration phase produces Calcium-Silicate-Hydrate (C-S-H) gel. C-S-H is formed by hydration of alite (tricalcium silicate or Ca3SiO4) and belite (Ca2SiO5). It can occupy more than 60% of the total volume of hydration products and has a variable composition. Thus, cement properties (strength, durability, conductivity…) will depend on C-S-H gel structure and composition.
Portlandite’s chemical composition is Ca(OH)2. Its share to the paste is almost 20 % by volume, formed by the hydration of alite and belite. Portlandite is a crystalline component with a hexagonal prism shape, responsible for the development of early age strength of fresh cement paste along with C-S-H gel.
The structure of C-S-H gel has been a subject of investigation over the last few decades, and significant advancements had being made employing both experimental tools and simulation studies. C-S-H has been found to have a poorly crystalline structure at the molecular level, often considered to be a defective tobermorite-like one.
Atomistic simulations are known to be a powerful tool in order to comprehend the intricate relationship between electron and hole transport in the context of thermal conductivity and thermoelectric properties. But they are rarely used in cement-based materials. Now, a new study 1 precisely aims to evaluate and optimize the thermoelectric efficiency of portlandite and tobermorite (mineral analogs of CSH gel nanostructure). The methodology is based on the density functional theory approach together with GW-quasiparticle and Boltzmann transport equation methods.
The results show, as could be expected, that the undoped minerals have low thermal conductivity. However, both the Seebeck coefficient and the electrical conductivity can be dramatically increased by appropriate carrier doping. ZT of portlandite exhibits improvement when subjected to p-type doping, leading to Z = 0.6 at 650 K. The same value is achieved with the introduction of n-type doping, the difference being in the hole carrier concentration. The thermoelectric characteristics of tobermorite-11 demonstrate a significant improvement with the p-type doping, with ZT = 0.79 at a temperature of 600 K.
These values confirm that there are still much promising prospects for enhancing the characteristics of concrete materials for energy harvesting.
Author: César Tomé López is a science writer and the editor of Mapping Ignorance
Disclaimer: Parts of this article may have been copied verbatim or almost verbatim from the referenced research paper/s.
References
- Ridwan O. Agbaoye, Jozef Janovec, Andrés Ayuela, Jorge S. Dolado (2024) Thermoelectric properties of the main species present in Portland cement pastes Cement and Concrete Research doi: 10.1016/j.cemconres.2024.107587 ↩