The dihydrogen activation and its splitting at single non-metal centers
The role of molecular hydrogen (dihydrogen) in reactions of organic and inorganic chemistry is very well known 1. One can mention numerous reactions playing the key role in biochemistry, like for example processes in microorganisms2, or the commonly known addition of H2 to organic compounds. It is worth to mention that dihydrogen as an alternative to traditional sources of energy is additionally a subject of numerous investigations 3. The latter is connected with the topic investigated extensively in recent years – it is the hydrogen storage 4; the molecular hydrogen is often routinely used in the industry but the cost of its transportation and storage is still very high.
That is why such processes as the activation of dihydrogen, its cleavage and next the reuniting of the molecular hydrogen are very important since they are strongly connected with the industrial modes of its use as a source of energy. The H2 activation at transition metal centers is analyzed most often56. However, the latter processes do not seem to be convenient for the industry where light materials occupying a small volume are preferred for the storage and transportation of dihydrogen. Hence non-metals to activate dihydrogen seem to be promising materials for the potential use in industry. However, studies on a role of non-metals for the molecular hydrogen cleavage are very rare. One can mention the theoretical study on the splitting of dihydrogen and ammonia by nucleophilic activation at a single carbon center of singlet carbenes 7. The singlet carbenes possess a lone pair of electrons and an accessible vacant orbital thus they can mimic the behavior of the transition metal centers. However the most important is that due to their electronic structure they have the dual character since they can act as the Lewis base and as a Lewis acid simultaneously.
Another theoretical study analyzes other non-metals, such as sulfur, phosphorus, and bromine as potential centers to activate and cleavage dihydrogen 8. Simple species containing non-metal centers, i.e. PFH2, SFH, AsFH2, SeFH, BrF3, PF(CH3)2 and AsF(CH3)2 (MP2/aug-cc-pVTZ calculations were performed) were considered as possible sites to activate dihydrogen. This is worth mentioning that all centers considered possess the dual acid-base character. For example, the sulfur center in the SFH molecule possesses the regions of the positive electrostatic potential (EP) in elongations of the H-S and F-S bonds to this center which are named as σ-holes 9 (see the previous article titled σ-hole bond as a preliminary stage of SN2 reaction in Mapping Ignorance where σ-hole concept is described) and the regions of negative EP attributed to the lone electron pairs situated outside of the SFH molecule plane (see Fig. 1a).
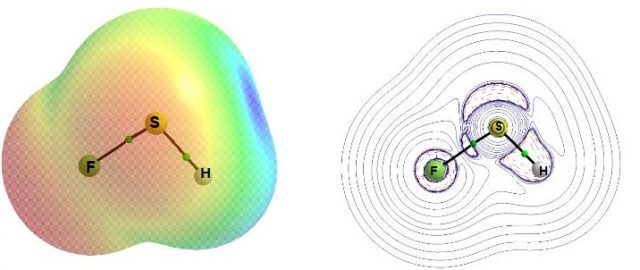
This is interesting that also dihydrogen is characterized by the dual Lewis acid-base properties (Fig. 2). The negative EP region is connected here with the -bond electrons while the positive EP is observed in elongations of the H-H bond, at both edges (see Fig. 2a). The map of the laplacian of the electron density confirms the latter findings since there is the broad region of the negative laplacian around the H-H σ-bond while thin regions of the negative laplacian in elongations of this bond are observed. This is why the H-nuclei charges outweigh the depleted regions of the electron charge at edges of H-atoms which results in the positive EP values.
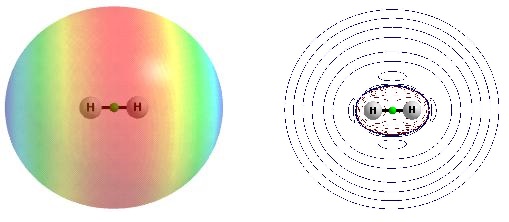
The following question arises; which properties, Lewis acid or Lewis base, of the non-metal center, play the crucial role in the activation of dihydrogen? Fig. 3 shows the SFH-H2 complex where the σ-bond of dihydrogen is situated approximately in the elongation of the F-S bond of the SFH molecule what may suggest that the positive EP region of sulfur (σ-hole) interacts with the negative EP region of H2 (σ-electrons); thus the S-centre plays the role of the Lewis acid while dihydrogen acts as the Lewis base here. Slightly different situation is observed for the PF(CH3)2-H2 complex where the P-centre is directed to one of H-atoms of dihydrogen and where the influence of the lone electron pair (the Lewis base properties) of phosphorus may be expected (Fig. 4).
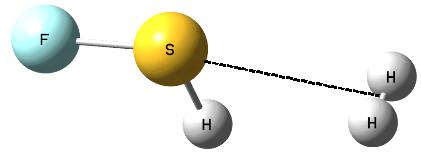
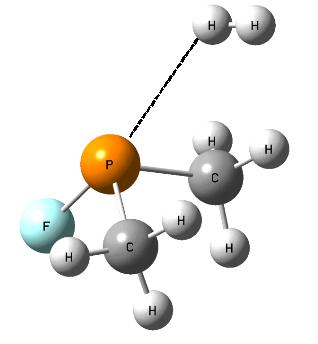
The orbital-orbital interactions were analyzed for the complexes of non-metal species with dihydrogen and it was found that for all of them two interactions are the most important ones; i.e. σ(H2) ® σ*(Z-F) and n(Z) ® σ*(H2) overlaps [8]; where Z means the non-metal centre (Z = S, Se, P, As and Br), s(H2) designates the H-H bond orbital; n(Z) is the lone electron pair orbital (of the Z non-metal centre), σ*(Z-F) is the antibonding orbital of Z-F bond while σ*(H2) is the antibonding orbital of the H-H bond. The first interaction, s(H2) ® σ*(Z-F), corresponds to the Lewis base properties of dihydrogen and the Lewis acid properties of the non-metal and vice versa, the second interaction, n(Z) ® σ*(H2) corresponds to the Lewis base properties of non-metal and the Lewis acid properties of dihydrogen. For all complexes analyzed [8] the duel character of the non-metal center is observed since both interactions exist, and similarly dihydrogen in those complexes reveals the dual character. Sometimes the Lewis acid character of the Z-center is stronger (SFH-H2 complex, Fig. 3), sometimes the Lewis base properties are stronger for the Z-centre (PF(CH3)2-H2 complex, Fig. 4).
The interactions in complexes mentioned above lead to the splitting of dihydrogen and to its addition to the Z-centre. Figure 5 presents the product of such addition for the PF(CH3)2-H2 reaction. This is interesting that all addition reactions for the non-metal complexes with dihydrogen considered in the recent study [8] are endothermic in the gas phase (in endothermic process the system absorbs energy from its surroundings), and the potential barrier heights for those reactions are relatively high since they are situated between 24 and 59 kcal/mol. The lowest potential barrier height of 24 kcal/mol was observed for the SFH + H2 SFH3 reaction. And this is why this reaction was analyzed in detail in different solvents [8].
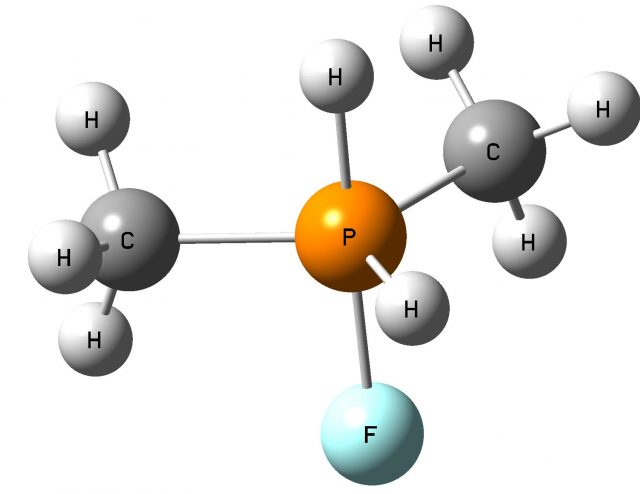
It was found that that the SFH + H2 SFH3 addition process is endothermic in the gas phase and in non-polar solvents such as benzene and cyclohexane while it is exothermic (in exothermic reaction the system releases energy by light or heat) in water and ethanol polar solvents.
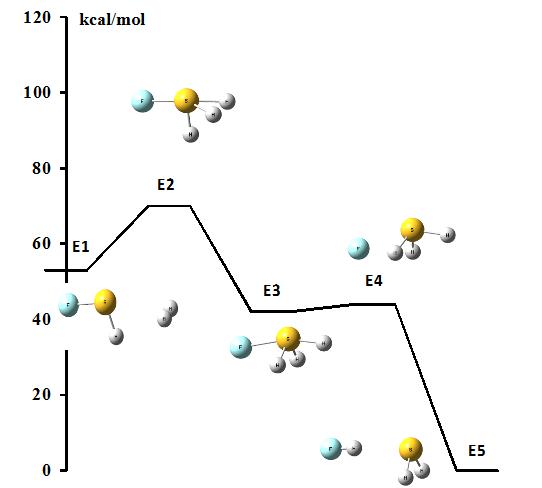
Figure 6 presents the reaction path where the interaction in the SFH-H2 complex (E1) leads to the addition process and the SFH3 product (E3) through the transition state (E2). Next the SFH3 may be decomposed into the H2S and HF molecules (E5). The latter complex (E5), linked through the F-H…S hydrogen bond, corresponds to the global energetic minimum what means that it is the most stable system among all configurations of the reaction path. This is not convenient for the industrial projecting of the hydrogen storage process since the reuniting of molecular hydrogen (for E1) from the E5 state is costly energetically. The H2 reuniting is more probable from the E3 energetic minimum. However the potential barrier height for the SFH3 H2S + HF reaction is very low (0.2-0.3 kcal/mol in polar solvent, look at Fig. 6 where the transition state E4 is only slightly above the SFH3 minimum – E3) what means that the E3 state corresponding to the SFH3 species may be difficult to preserve. This is why the activation and splitting of dihydrogen at non-metal single centers need additional numerous studies.
References
- ¨March´s Advanced Organic Chemistry¨. M.B.Smith, J.March, Sixth Ed. John Wiley & Sons, Inc., Hoboken, New Jersey, 2007 ↩
- ¨Reactions with Molecular Hydrogen in Microorganisms: Evidence for a Purely Organic Hydrogenation Catalyst¨. R.K.Thauer, A.R.Klein, G.C.Hartmann, Chem.Rev. 1996, 96, 3031-3042. ↩
- ¨Hydrogen as a fuel for today and tomorrow: expectations for advanced hydrogen storage materials/systems research¨. K. Hirose, Faraday Discuss. 2011, 151, 11-18. ↩
- ¨High capacity hydrogen storage materials: attributes for automotive applications and techniques for materials discovery¨. J.Yang, A.Sudik, C.Wolverton, D.J.Siegel, Chem.Soc.Rev. 2010, 39, 656-675. ↩
- ¨Breaking the H2 Marriage and Reuniting the Couple¨. G.J. Kubas, Science 2006, 314, 1096-1097. ↩
- ¨Activation of dihydrogen and coordination of molecular H2 on transition metals¨. G.J. Kubas, J.Organomet.Chem. 2014, 751, 33-49. ↩
- ¨Facile Splitting of Hydrogen and Ammonia by Nucleophilic Activation at a Single Carbon Center¨. G.D. Frey, V. Lavallo, B. Donnadieu, W.W. Schoeller and G. Bertrand, Science 2007, 316, 439-441. ↩
- ¨Cleavage of hydrogen by activation at a single non-metal centre – towards new hydrogen storage materials¨. S.J.Grabowski, Phys.Chem.Chem.Phys. 2015, 17, 13539-13546. DOI: 10.1039/C5CP00219B ↩
- ¨Halogen bonding and other -hole interactions: a perspective¨. P.Politzer, J.S.Murray, T.Clark, Phys.Chem.Chem.Phys. 2013, 15, 11178-11189. ↩