Aerogen bonding as a new kind of intermolecular interaction
What more can be learnt from the chemistry of noble gases?
Noble gases, the members of group 18 of the periodic table of elements (in previous terminology – group VIII) are known as monoatomic gases. The latter property is the result of their very low chemical reactivity thus, they do not form bonds between themselves. The extremely low chemical reactivity of those gases may be explained by their atomic structures. It is commonly known that their outer shell of valence electrons is fully occupied and therefore very stable. As a result, noble gas atoms hardly accept or donate electrons; in other words, they hardly react as Lewis acids or Lewis bases, respectively. Consequently, only a few hundreds noble gas compounds have been formed; mostly the species of xenon (Xe). However chemistry of noble gases is growing rapidly thus, one may expect many uses of their compounds; similarly, as numerous applications of pure noble gases have been already reported (for example in medicine).
The chemistry of noble gases concerns their reactivity and consequently also the properties of compounds formed, where mainly covalent in nature bonds between noble gas centres and other more reactive atoms are important. However very recently few studies were performed on energetically favourable intermolecular interactions between the covalently bonded atoms of Group 18 and the Lewis base centres 12. Such interactions were named as aerogen bonds because aerogens is the other name of noble gases. The noble gas centres play a role of Lewis acids in aerogen bonds thus they interact with Lewis bases (lone electron pairs, anions or any other electron donating centres).
The following question arises: why the aerogen atom may act as the Lewis acid, i.e. as the electron accepting centre? This is because for numerous compounds the regions of the positive electrostatic potential (EP) at the aerogen centre exist. Two aerogen species, i.e. XeO3 and XeF2O were analyzed in detail [1] and such positive EP regions were found at xenon centre (Fig. 1). The XeO3 molecule is characterized by the trigonal pyramid shape (C3v symmetry) with the lone electron pair situated at the 3-fold symmetry axis of the molecule. This is why for the wide region of the positive EP of above 50 kcal/mol at the Xe-centre three equivalent EP maxima which avoid the position of a lone pair are observed, approximately in the elongations of O=Xe bonds. Those EP maxima are equal to ~58 kcal/mol.
Such regions of the positive EP for other compounds of the elements of groups 14-17 of the periodic table were named in literature as the σ-holes 3. Their existence was explained by the participation of electrons of the centre considered in σ-bonds what results in the depletion of the electron density in regions being elongations of these bonds. Hence, those regions are often characterized by the positive EP (see article ¨σ-hole bond as a preliminary stage of SN2 reaction¨).
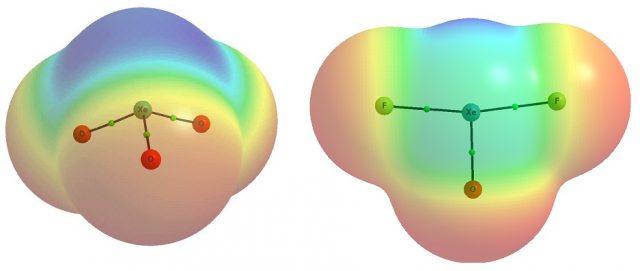
For the T-shaped XeF2O molecule (C2v symmetry) the single EP maximum of 90 kcal/mol exists (Fig. 1) in the extension of the O=Xe bond. The location of the EP maxima has important consequences. The complexes of the mentioned here XeO3 and XeF2O species with different Lewis bases are observed; the corresponding calculations at the MP2/aug-cc-pVTZ level were performed. It was found that the Lewis base centres are located exactly opposite to the σ-holes; it means in the extensions of O=Xe bonds. The interaction energies of these two xenon moieties with neutral species, NH3 and CH3CN, playing the role of Lewis bases through the nitrogen centres, are situated between -8 and -13 kcal/mol. These are not negligible interactions since the interaction energy for the water dimer linked through the medium in strength O-H…O hydrogen bond amounts ~ -5 kcal/mol. And the crucial role of water in life processes due to these H-bond interactions is very well known 4. This is worth to mention that the interaction energies in complexes of XeO3 and XeF2O with Cl– and Br– anions are situated in the range between -32 and -40 kcal/mol. Such strong interactions are very well known in the literature as being supported by charge; positive or negative charge assistance is observed in numerous interactions [4]. The similar complexes of the lighter Ar and Kr noble gas elements, i.e. the complexes of ArO3 and KrO3 with NH3 and CH3CN were analyzed. The interactions for those complexes are weaker than for the xenon analogues, but not negligible, approximately between -6 and -8 kcal/mol [1]. These interactions were analyzed in detail [1] and they were classified as a special kind of the σ-hole bonds, i.e. as the aerogen bonds, similarly as interactions in complexes of xenon.
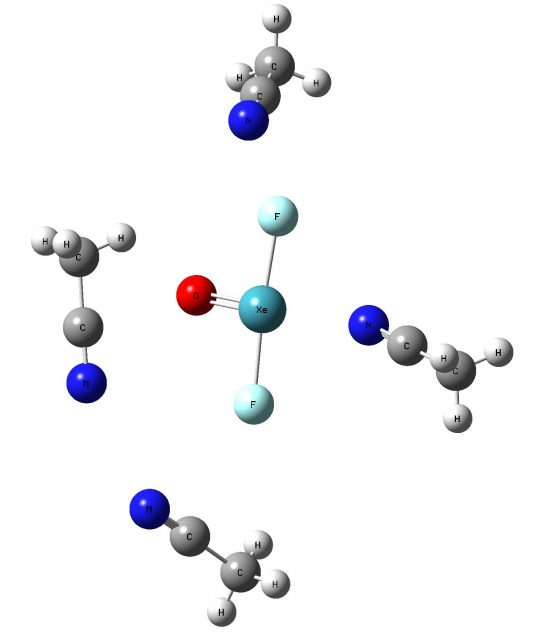
One can see that the noble gas compounds may interact strongly through the σ-holes of the aerogen centres with the Lewis bases thus, they could be important in numerous processes and reactions. For example, they should play a meaningful role in the arrangement of molecules in crystals. Two X-ray crystal structures, where such interactions exist, have been presented recently [1]; the crystal structure of the mentioned above XeO3 compound, where there are the Xe…O intermolecular distances of about 2.8 Å, much less than the corresponding sum of van der Waals radii (the O and Xe radii are equal to 1.52 and 2.16 Å, respectively, according to the Bondi scale5). It is commonly accepted that the inter-atomic contacts much smaller than the corresponding van der Waals radii sum is an evidence of the stronger interactions, where not only dispersive forces take place, but also the electrostatic contribution is important, as well as the charge transfer and polarization effects play the crucial role 6. Another structure of mixed crystal; XeF2O.CH3CN shows the Xe…N distances of 2.81 Å (Fig. 2). The nitrogen van der Waals radius is equal to 1.55 Å [5], thus also here strong stabilizing interactions are observed since the corresponding radii´ sum is equal to 3.71 Å. One can see that the XeF2O molecule in that crystal structure is coordinated by four CH3CN molecules. In addition to the above-mentioned Xe…O contact there are two F…C interactions (2.9-3 Å) and one O…C contact (3.1 Å).
There are not experimental evidence in crystal structures of the existence of aerogen bonds for non-xenon noble gas compounds. However, several crystal structures with the xenon – Lewis base centre interactions (aerogen bonds) may be presented. Fig. 3 shows the C6H5Xe…CH3CN and C6H5Xe…B(CN)4 complexes existing in the crystal structures (results are taken here from the Cambridge Structural Database [7]) where there are short Xe…N contacts, of 2.61 and 2.71 Å, respectively.
Fig. 4 shows the fragment of the other interesting crystal structure. The C20F14O4Xe2 molecule is surrounded by the C3F6OH2 ligands. On one hand the xenon atoms for the former species may be treated as divalent ones but, on the other hand, the whole C20F14O4Xe2 system may be considered as two C6H5Xe+ cations interacting through the Xe…O links with the [C6F4(COO)2]2- anion. Note that the Xe-C and Xe-O distances are equal here to 2.1 and 2.5 Å, respectively; especially the second distance is very close to the Xe…O and Xe…N distances of 2.7-3.0 Å presented earlier here for the other crystal structures.
Summarizing, one can see that the aerogen bond described in recent studies is classified as a kind of σ-hole bond [1,2]. The latter is the interaction between the atomic region characterized by the depletion of the electron charge and consequently by the positive electrostatic potential thus playing the role of the Lewis acid with the Lewis base centre. This interaction for noble gas complexes is strong enough to initiate different chemical processes and reactions thus probably will be a subject of numerous studies in the near future.
References
- A Bauzá, A. Frontera, Aerogen Bonding Interaction: A New Supramolecular Force? Angew. Chem. Int. Ed. 2015, 54, 7340-7343. ↩
- A Bauzá, A. Frontera, π-Hole aerogen bonding interactions. Phys. Chem. Chem. Phys. 2015, 17, 24748-24753. ↩
- A. Bundhun, P. Ramasami, J. S. Murray, P. Politzer, Trends in σ-hole strengths and interactions of F3MX molecules (M = C, Si, Ge and X = F, Cl, Br, I). J.Mol.Model. 2012, 19, 2739–2746. ↩
- Hydrogen Bonding – New Insights, Ed. S.J.Grabowski, Springer, 2006. ↩
- A. Bondi, van der Waals Volums and Radii. J. Phys. Chem. 1964, 68, 441-451. ↩
- S.J.Grabowski, What is the Covalency of Hydrogen Bonding. Chem. Rev. 2011, 111, 2597-2625. ↩